The prognostic importance of the heart function in sepsis and septic shock: a Dedalus or a window of opportunities?
Sepsis is an infection-related clinical condition characterized by severe systemic inflammation, potentially evolving in multi-organ failure through a number of pathophysiological mechanisms. While sepsis is a relatively infrequent disease, it is a world-wide growing and challenging medical issue (1-3), both due to high mortality rate in the short and mid-term follow-ups, and to high costs of medical management (4,5). Hemodynamic instability and sustained hypotension with systemic hypoperfusion not responding to blood volume expansion are the hallmarks of the sepsis-related shock, or septic shock. In sepsis, refractory cardiovascular failure is a frequent cause of fatal events, and myocardial injury can be found in approximately 50% of the patients in necropsy findings (6). Therefore, one cannot infer that sepsis or septic shock is equivalent to myocardial dysfunction.
In 1984, in a group of septic patients, Parker et al. (7) reported increased left ventricular (LV) end-diastolic volume and reduced LV ejection fraction (EF) in survivors while the non-survivors showed normal LV volumes and EF, and lower stroke volume, up to fatal events. Increased LV volumes and reduced EF were reversible in survivors to sepsis. A few years later, using radionuclide ventriculography, Parker et al. (8) reported that, in patients with sepsis, right ventricular (RV) systolic dysfunction can be as frequent as LV systolic dysfunction. They also found that reversible RV chamber dilatation and systolic dysfunction characterized survivors rather than non-survivors. Overall, those early investigations on heart systolic function in sepsis and septic shock described the paradox of worse prognosis in those who had early normal systolic chamber function.
Subsequent echocardiographic studies (Tables 1,2) on heart structure function failed to confirm relationships of LV and RV dimensions and systolic function with prognosis in sepsis (9). Actually, echocardiographic studies revealed that a significant proportion of non-survivors to sepsis or septic shock actually showed early-depressed LV EF (10,11). With regard to RV function and prognosis in sepsis, echocardiographic studies (12) did not confirm the initial report by Parker et al. For instance, Landesberg et al. (13) found that RV dilation was independently associated with mortality, altogether with greater Acute Physiology and Chronic Health Evaluation (APACHE) score, impaired renal function and LV diastolic dysfunction. Further studies in sepsis questioned the prevalence and the prognostic significance of impaired RV systolic function as compared to LV systolic dysfunction (14). The lack of consistency in results from different studies (Tables 1,2) have raised more skepticism on the real prognostic impact of myocardial function in septic patients. As a consequence, a generalized opinion has been growing that cardiovascular involvement in the septic process may be essentially described by reporting mean arterial pressure and the ongoing treatment with vasoactive medications, to account for cardiovascular impairment as one of the potential multi-organ failure in sepsis (Sequential Organ Failure Assessment, SOFA). The recently published Surviving Sepsis Campaign (SSC) considered echocardiography to assess fluid-responsiveness to fluid bolus, recognized the presence of a sepsis-related myocardial dysfunction by means of EF, and stated (cit.) “measurement of cardiac output along with a measure of the adequacy of perfusion is preferable” over echocardiography to identify patients who might benefit from dobutamine administration.
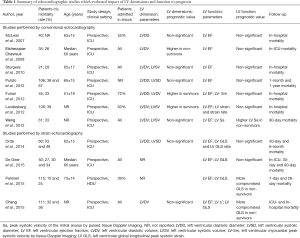
Full table
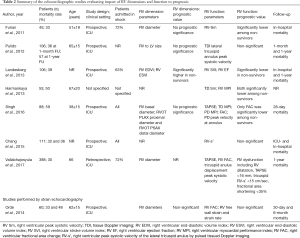
Full table
Nonetheless, the issue of a potential link between heart systolic function and prognosis in sepsis or septic shock was raised brought up by a recent retrospective investigation from the Mayo Clinic (15), reporting that RV systolic dysfunction may be common and prognostically relevant in long-term follow-up in septic patients. The study provided interesting data in 388 patients of whom 55% showed RV dysfunction (16) (i.e., tricuspid anulus plane systolic excursion (TAPSE) below 16 mm or its peak velocity below 15 cm/sec or RV fractional area change below 35%). Of the study sample, 26% showed isolated RV dysfunction, i.e. without associated LV dysfunction defined based on LV EF <50% (17). As reported (15), reduced TAPSE (38%) or enlarged RV (31%) were the main contributors to the definition of RV dysfunction, whereas 1 in 5 patients studied showed simultaneously both RV abnormalities. Systemic hemodynamics did not differ significantly among the septic patients stratified based on the absence or the presence of RV systolic dysfunction, independent to the presence or the absence of LV systolic dysfunction. Of note, LV stroke index was on average 40 mL/m2 with RV dysfunction, roughly comparable to the mean stroke index measured in septic patients without RV dysfunction. Cardiac index was 3.5 l/min/m2 compared to the 3.8 (interquartile rage 2.8–4.1) L/min/m2 in patients without RV dysfunction, and was lowest in those with LV dysfunction in line with lowest stroke index mean values. LV preload was also comparable among patients stratified according to RV/LV systolic dysfunction, as the peak velocity of the mitral E wave (i.e., the early LV filling wave), and the ratio between the peak velocity of the mitral E wave and the peak velocity of the early diastolic displacement of the mitral annulus (so called é), were both comparable among the group of patients independent to ventricular dysfunction. The peak velocity of the mitral E wave represents the instantaneous peak gradient between the left atrium and the LV in proto-diastole, so that, mitral E wave peak velocity can be assumed as an indirect measure of LV preload, determined by the active LV suction and the passive effect of left atrium blood volume. All groups shared a high percent of patients with shock (80% among those with isolated RV dysfunction, 63% among those without RV dysfunction, 72% among those with both RV and LV dysfunction); SOFA as well as APACHE-III score, blood lactate, mean airway pressure applied, as well as total nor-epinephrine and crystalloid used in 24h, were all comparable among the groups of patients. Therefore, Vallabhajosyula et al. (15) described patients differing based on load-dependent indicators of RV and LV systolic chamber function but not in terms of hemodynamics. Ultimately, those data support the hypothesis that subjects with reduced LV or RV systolic function at comparable central hemodynamics may have significant myocardial dysfunction, and worse prognosis at one-year follow-up, independently of APACHE-III score, and with no prognostic contributions from troponins, lactates, LV dysfunction, age, status of shock, and mechanical ventilation. Survival curves did not distinguished patients with versus without RV dysfunction in the first month of observation, whereas curves diverged by the second month of observation, and remained separated up to the 12-month follow-up. The point of long-term survival in sepsis is important. However, for a clinical dynamic condition with a high risk of in-hospital death, such as sepsis and septic shock, short-term predictors of untoward events are potentially more relevant for clinical-decision making than those emerging only in the long-term.
Because investigations on heart chambers structure and function as prognostic indicators in sepsis and septic shock provided inconsistent results, a parallel research focused on laboratory indicators of myocardial injury, myocardial stress and severity of inflammation, such as troponins, natriuretic peptides, and cytokines, for risk stratification, monitoring the process, predicting prognosis. Several experimental studies documented the presence of circulating cytokines, which induce myocardial contractile impairment via mechanisms such as nitric oxide overproduction or calcium ion leakage from the sarcoplasmic reticulum. The issue of circulating myocardial depressant factor(s) emerged (18) as pathophysiological mechanism and target, even though research has been inconclusive (19,20). In fact, a clear correlation between circulating cytokines and LV EF as marker of LV systolic function was substantially inconsistent (21). Furthermore, the time-course of the cardiac-specific troponins has been considered as possible surrogate for the relation between the extent of myocardial damage, risk stratification and prognosis in sepsis. However, because different processes may end with myocardial damage, it remains to be clarified whether the mechanisms of cytolysis or the cytolysis in itself is relevant in sepsis. Troponin release from cardiac myocytes is supposed to occur by mechanisms such as imbalanced myocardial oxygen supply-demand even in absence of critical coronary stenoses, alteration of oxygen extraction and cellular utilization, inflammation-induced apoptosis, and increased cellular permeability. Additional contribution to myocardial injury in sepsis may be related to increased endogenous or exogenous catecholamines. Myocardial stress with myocardial dysfunction in sepsis may explain in part the increased levels of N-terminal-brain natriuretic peptide (NT-proBNP), whereas a contribution may come from reduced inactivation of NT-proBNP in acute inflammation (13,22-24). Troponins and NT-proBNP both elevated were proven to be associated with worse prognosis and, in a less consistent manner, with the presence of myocardial systolic or diastolic dysfunction in sepsis. However, because cytolyses are invariably elevated in sepsis, and levels of specific markers of inflammation as well as of myocardial necrosis are highly variable intra-patients and between-patients, relationships of plasma levels of those biomarkers with heart function and prognosis in sepsis has not emerged as strong as hypothesized, and the use of those bio-markers have not been turned to be useful in bedside decision-making processes (25-27). Therefore, the attention has remained high on heart structure and function for risk stratification and monitoring disease evolution in sepsis.
As a general concept, EF is the percent of end-diastolic volume ejected in systole, numerically computed as ratio between stroke volume and end-diastolic volume, representing a measure of chamber systolic function. RV fractional area change and TAPSE approximates RV EF, explored today in clinical research by real-time 3D echocardiography (28). Because LV EF, as well as TAPSE or RV area fractional shortening, are sensitive to preload and afterload, they cannot provide reliable information on myocardial systolic performance. For instance, a 50% EF may result from a stroke volume of 120 mL divided by 240 mL of end-diastolic volume, or the result of 60 mL of stroke volume on 120 mL of end-diastolic volume. Moreover, for comparable EF values, as in the latter case, myocardial systolic performance differs according to the La Place’s Law, because afterload is higher with greater systolic blood pressure and larger end-systolic diameter, but is lower with thicker wall in systole (29-31). Sepsis is a clinical scenario in which loading conditions and myocardial contractility can be largely variable intra-patient at different times, as well as between-patients. Therefore, a shift in the identification LV function parameters is needed in order to explore whether in sepsis assessment of myocardial performance is useful for triaging patients, following treatments efficacy, and predict prognosis.
Novel technologies in echocardiography have boosted new research in the field of myocardial dysfunction in sepsis and septic shock (Tables 1,2) (32-38). Shifting from investigating chamber function to myocardial contractility by minimally operator-dependent techniques, bedside-ready, is the key-element for employing those technologies in patients with sepsis and septic shock. One of the new echocardiographic methods of assessment of ventricular systolic and diastolic function is based on tracking two-dimensional myocardial speckles to describe myocardial deformation through the cardiac cycle (39). The myocardial deformation thought the cardiac cycle is defined strain, which can be measured during systole as well as diastole, and can be described as deformation in the dimension of time, therefore called strain-rate. With a sufficient number of speckles traced, semi-automatic procedures allow operators to obtain strain and strain-rate even bed-side. In addition, the contribution of the performance of different myocardial layers to the global function may be explored, granted a sufficient number of speckles are traced in the “space” over time. The global longitudinal peak systolic strain (GLS) is the indicator of systolic myocardial function most commonly used is clinical practice. GLS is significantly less load-independent compared to LV EF or TAPSE (40). Procedures to obtain sections of the heart useful for quantitative analysis (i.e., strain and strain-rate assessments) do not differ substantially from those usually employed in daily practice for heart imaging by ultrasound. As quality of imaging is a key-factor for assessments of EF, TAPSE and heart volumes, a good quality of images of the heart is important for strain and strain rate assessments. Such a technology is now available on portable echocardiographic machines, giving the possibility of measuring GLS bedside. GLS should most help in risk stratification of patients beyond load-dependent measure of chamber systolic function, to resolve the initial paradox of worse prognosis in septic patients with preserved LV EF as described by Parker et al. (7). There is a possibility that GLS may describe unappreciated myocardial dysfunction in septic patients with normal or nearly normal EF or TAPSE. The relationship between LV GLS and EF was reported to be heteroscedastic toward higher values of both indicators, so that that for EF >30%, information from LV GLS and from LF EF do no overlap, and LV GLS may provide information on short-term prognosis independent and beyond LV EF in septic patients (41). GLS of the RV free wall was also reported to be prognostically relevant in the short term in sepsis and septic shock (42). Actually, in sepsis and septic shock myocardial systolic function predicted prognosis independent to age and sepsis severity based on SOFA, in the very short term (43). Several investigations have demonstrated that GLS was able to identify systolic dysfunction in a significantly higher proportion of septic patients as compared to conventional echocardiography. Nevertheless, the association of impaired LV GLS with adverse prognosis in sepsis may be relatively weak (42,44-46). Investigations on potential contribution of RV GLS to prognosis in sepsis is even more problematic, first of all because RV free wall is thin, and consequently the number of speckles may be relatively low for reliable function quantification.
Notwithstanding, the idea that sepsis could be phenotyped according to GLS is seducing. Fatal events in sepsis in the short term appear to be related to LV systolic function more strongly than the dramatic condition of shock in itself (Figure 1, data unpublished, abbreviations as in text). Nevertheless, research in the field cannot be considered conclusive, as the use of GLS for clinical decision making in sepsis is far from being established. Correlates and determinants of GLS in sepsis are still under-investigations. Studies using both hemodynamic and heart dysfunction phenotypization in sepsis and septic shock are lacking. To date, risk stratification in sepsis and septic shock remains a challenging task. Such a research field requires a strong joint-invention between cardiology and emergency medicine for the future.
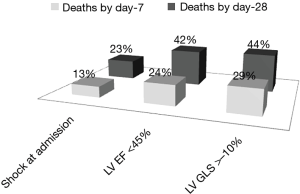
Acknowledgements
None.
Footnote
Conflicts of Interest: The authors have no conflicts of interest to declare.
References
- Angus DC, Linde-Zwirble WT, Lidicker J, et al. Epidemiology of severe sepsis in the United States: analysis of incidence, outcome, and associated costs of care. Crit Care Med 2001;29:1303-10. [Crossref] [PubMed]
- Martin GS, Mannino DM, Eaton S, et al. The epidemiology of sepsis in the United States from 1979 through 2000. N Engl J Med 2003;348:1546-54. [Crossref] [PubMed]
- Martin GS. Sepsis, severe sepsis and septic shock: changes in incidence, pathogens and outcomes. Expert Rev Anti Infect Ther 2012;10:701-6. [Crossref] [PubMed]
- Sakr Y, Elia C, Mascia L, et al. The influence of gender on the epidemiology of and outcome from severe sepsis. Critical Care 2013;17:R50. [Crossref] [PubMed]
- Dombrovskiy VY, Martin AA, Sunderram J, et al. Rapid increase in hospitalization and mortality rates for severe sepsis in the United States: A trend analysis from 1993 to 2003. Critical Care Medicine 2007;35:1244-50. [Crossref] [PubMed]
- Torgersen C, Moser P, Luckner G, et al. Macroscopic postmortem findings in 235 surgical intensive care patients with sepsis. Anesth Analg 2009;108:1841-7. [Crossref] [PubMed]
- Parker MM, Shelhamer JH, Bacharach SL, et al. Profound but reversible myocardial depression in patients with septic shock. Ann Intern Med 1984;100:483-90. [Crossref] [PubMed]
- Parker MM, McCarthy KE, Ognibene FP, et al. Right ventricular dysfunction and dilatation, similar to left ventricular changes, characterize the cardiac depression of septic shock in humans. Chest 1990;97:126-31. [Crossref] [PubMed]
- Huang SJ, Nalos M, McLean AS. Is early ventricular dysfunction or dilatation associated with lower mortality rate in adult severe sepsis and septic shock? A meta-analysis. Crit Care 2013;17:R96. [Crossref] [PubMed]
- Vieillard-Baron A, Caille V, Charron C, et al. Actual incidence of global left ventricular hypokinesia in adult septic shock. Crit Care Med 2008;36:1701-6. [Crossref] [PubMed]
- Bouhemad B, Nicolas-Robin A, Arbelot C, et al. Acute left ventricular dilatation and shock-induced myocardial dysfunction. Crit Care Med 2009;37:441-7. [Crossref] [PubMed]
- Furian T, Aguiar C, Prado K, et al. Ventricular dysfunction and dilation in severe sepsis and septic shock: Relation to endothelial function and mortality. J Crit Care 2012;27:319.e9-15. [Crossref] [PubMed]
- Landesberg G, Gilon D, Meroz Y, et al. Diastolic dysfunction and mortality in severe sepsis and septic shock. Eur Heart J 2012;33:895-903. [Crossref] [PubMed]
- Pulido JN, Afessa B, Masaki M, et al. Clinical spectrum, frequency, and significance of myocardial dysfunction in severe sepsis and septic shock. Mayo Clin Proc 2012;87:620-8. [Crossref] [PubMed]
- Vallabhajosyula S, Kumar M, Pandompatam G, et al. Prognostic impact of isolated right ventricular dysfunction in sepsis and septic shock: an 8-year historical cohort study. Ann Intensive Care 2017;7:94. [Crossref] [PubMed]
- Rudski LG, Lai WW, Afilalo J, et al. Guidelines for the echocardiographic assessment of the right heart in adults: a report from the American Society of Echocardiography endorsed by the European Association of Echocardiography, a registered branch of the European Society of Cardiology, and the Canadian Society of Echocardiography. J Am Soc Echocardiogr 2010;23:685-713. [Crossref] [PubMed]
- Lang RM, Badano LP, Mor-Avi V, et al. Recommendations for cardiac chamber quantification by echocardiography in adults: an update from the American Society of Echocardiography and the European Association of Cardiovascular Imaging. Eur Heart J Cardiovasc Imaging 2015;16:233-70. [Crossref] [PubMed]
- Parrillo JE, Burch C, Shelhamer JH, et al. A circulating myocardial depressant substance in humans with septic shock. Septic shock patients with a reduced ejection fraction have a circulating factor that depresses in vitro myocardial cell performance. J Clin Invest 1985;76:1539-53. [Crossref] [PubMed]
- Zanotti-Cavazzoni SL, Hollenberg SM. Cardiac dysfunction in severe sepsis and septic shock. Curr Opin Crit Care 2009;15:392-7. [Crossref] [PubMed]
- Rudiger A, Singer M. Mechanisms of sepsis-induced cardiac dysfunction. Crit Care Med 2007;35:1599-608. [Crossref] [PubMed]
- Landesberg G, Levin PD, Gilon D, et al. Myocardial Dysfunction in Severe Sepsis and Septic Shock: No Correlation With Inflammatory Cytokines in Real-life Clinical Setting. Chest 2015;148:93-102. [Crossref] [PubMed]
- Post F, Weilemann LS, Messow CM, et al. B-type natriuretic peptide as a marker for sepsis-induced myocardial depression in intensive care patients. Critical Care Medicine 2008;36:3030-7. [Crossref] [PubMed]
- Charpentier J, Luyt CE, Fulla Y, et al. Brain natriuretic peptide: A marker of myocardial dysfunction and prognosis during severe sepsis. Crit Care Med 2004;32:660-5. [Crossref] [PubMed]
- Turner KL, Moore LJ, Todd SR, et al. Identification of cardiac dysfunction in sepsis with B-Type Natriuretic Peptide. J Am Coll Surg 2011;213:139-46; discussion 146-7. [Crossref] [PubMed]
- Mehta NJ, Khan IA, Gupta V, et al. Cardiac troponin I predicts myocardial dysfunction and adverse outcome in septic shock. Int J Cardiol 2004;95:13-7. [Crossref] [PubMed]
- Tiruvoipati R, Sultana N, Lewis D. Cardiac troponin I does not independently predict mortality in critically ill patients with severe sepsis. Emerg Med Australas 2012;24:151-8. [Crossref] [PubMed]
- Zhang Z, Ni H, Lu B, et al. Changes in brain natriuretic peptide are correlated with changes in global end-diastolic volume index. J Thorac Dis 2013;5:156-60. [PubMed]
- Lang RM, Mor-Avi V, Sugeng L, et al. Three-dimensional echocardiography: the benefits of the additional dimension. J Am Coll Cardiol 2006;48:2053-69. [Crossref] [PubMed]
- Borow KM, Green LH, Grossman W, et al. Left ventricular end-systolic stress-shortening and stress-length relations in human. Normal values and sensitivity to inotropic state. Am J Cardiol 1982;50:1301-8. [Crossref] [PubMed]
- de Simone G, Devereux RB, Roman MJ, et al. Assessment of left ventricular function by the midwall fractional shortening/end-systolic stress relation in human hypertension. J Am Coll Cardiol 1994;23:1444-51. [Crossref] [PubMed]
- Russo C, Jin Z, Homma S, et al. Relationship of multidirectional myocardial strain with radial thickening and ejection fraction and impact of left ventricular hypertrophy: a study in a community-based cohort. Echocardiography 2013;30:794-802. [Crossref] [PubMed]
- McLean AS, Huang SJ, Hyams S, et al. Prognostic values of B-type natriuretic peptide in severe sepsis and septic shock. Crit Care Med 2007;35:1019-26. [Crossref] [PubMed]
- Etchecopar-Chevreuil C, François B, Clavel M, et al. Cardiac morphological and functional changes during early septic shock: a transesophageal echocardiographic study. Intensive Care Med 2008;34:250-6. [Crossref] [PubMed]
- Sturgess DJ, Marwick TH, Joyce C, et al. Prediction of hospital outcome in septic shock: a prospective comparison of tissue Doppler and cardiac biomarkers. Crit Care 2010;14:R44. [Crossref] [PubMed]
- Weng L, Liu YT, Du B, et al. The prognostic value of left ventricular systolic function measured by tissue Doppler imaging in septic shock. Crit Care 2012;16:R71. [Crossref] [PubMed]
- Chang WT, Lee WH, Lee WT, et al. Left ventricular global longitudinal strain is independently associated with mortality in septic shock patients. Intensive Care Med 2015;41:1791-9. [Crossref] [PubMed]
- Harmankaya A, Akilli H, Gul M, et al. Assessment of right ventricular functions in patients with sepsis, severe sepsis and septic shock and its prognostic importance: a tissue Doppler study. J Crit Care 2013;28:1111.e7-1111.e11. [Crossref] [PubMed]
- Singh S, Mohan S, Singhal R. Definitions for sepsis and septic shock. JAMA 2016;316:458. [Crossref] [PubMed]
- Shah AM, Solomon SD. Myocardial deformation imaging: current status and future directions. Circulation 2012;125:e244-8. [Crossref] [PubMed]
- Burns AT, La GA, D'hooge J, et al. Left ventricular strain and strain rate: characterization of the effect of load in human subjects. Eur J Echocardiogr 2010;11:283-9. [Crossref] [PubMed]
- Palmieri V, Innocenti F, Guzzo A, et al. Left ventricular systolic longitudinal function as predictor of outcome in patients with sepsis. Circ Cardiovasc Imaging 2015;8:e003865. [PubMed]
- Orde SR, Pulido JN, Masaki M, et al. Outcome prediction in sepsis: speckle tracking echocardiography based assessment of myocardial function. Crit Care 2014;18:R149. [Crossref] [PubMed]
- Innocenti F, Palmieri V, Guzzo A, et al. SOFA score and left ventricular systolic function as predictors of short-term outcome in patients with sepsis. Intern Emerg Med 2016. [Epub ahead of print]. [Crossref] [PubMed]
- Shahul S, Gulati G, Hacker MR, et al. Detection of Myocardial Dysfunction in Septic Shock: A Speckle-Tracking Echocardiography Study. Anesth Analg 2015;121:1547-54. [Crossref] [PubMed]
- De Geer L, Engvall J, Oscarsson A. Strain echocardiography in septic shock - a comparison with systolic and diastolic function parameters, cardiac biomarkers and outcome. Crit Care 2015;19:122. [Crossref] [PubMed]
- Dalla K, Hallman C, Bech-Hanssen O, et al. Strain echocardiography identifies impaired longitudinal systolic function in patients with septic shock and preserved ejection fraction. Cardiovasc Ultrasound 2015;13:30. [Crossref] [PubMed]
Cite this article as: Palmieri V, Innocenti F, Pini R. The prognostic importance of the heart function in sepsis and septic shock: a Dedalus or a window of opportunities? J Emerg Crit Care Med 2017;1:43.