Pediatric traumatic brain injury—a review of management strategies
Introduction
Traumatic brain injury (TBI) in children may result in death, permanent neurological deficit, and dependence on caregivers for all activities of daily living (1-3). TBI-related deaths range from 1.9 per 100,000 children aged 5 to 14 years old, to 4.3 per 100,000 children aged 0 to 4 years old in the United States in 2009–2010 (4). Recent studies found that children with severe TBI (5,6), those younger than 7 years of age (7), and those with severe concomitant injuries are at higher risk for mortality (8). Studies looking at long term outcomes in children found an association between previous TBI and a decreased ability in daily functioning (9), pronounced behavioral changes (10-12) and difficulty coping with mainstream academic demands (10). All these form a significant burden from a health economics perspective (13-15). Yet, TBI is under-recognized and under-studied, especially in many low- and middle-income countries (13,16).
When encountering a head-injured child, favorable neurological outcomes depend on prompt identification and treatment of raised intracranial pressure (ICP) together with swift and effective resuscitation.
In this narrative review, we aim to focus on the following areas in the acute management of pediatric TBI: (I) airway management with sedation and hyperventilation; (II) utility of hyperosmolar agents; (III) effect of hypothermia; (IV) glycemic control; (V) indications for neuroimaging; (VI) role of ICP monitoring, and (VII) indications for prophylactic anti-epileptics.
Materials and methods
We searched PubMed with the following MeSH search terms: brain injuries; child; hypnotics and sedation; hypocapnia; hypertonic solution, saline; hypothermia; glycemia and hyperglycemia; neuroimaging; ICP monitoring and anticonvulsants. Both original research and review articles that were relevant to each area of focus were included. We chose to exclude case reports and non-English articles. Regarding specific treatment strategies, we included relevant references from the adult TBI literature for discussion.
Results and discussion
Airway management, sedation and ventilation strategies
Prompt endotracheal intubation in an unconscious TBI patient [Glasgow coma scale (GCS) score ≤8] or one with rapidly deteriorating GCS allows for airway control, concomitant management of raised ICP and prevents hypoxemia, an important cause of secondary injury to the brain (17). When managing the airway, concurrent decisions have to be made to select an appropriate sedative agent. The appropriate choice and dosage of sedative agents facilitate airway intervention (18), while maintaining both mean arterial pressure (MAP) and cerebral perfusion pressure (CPP) (17,19).
Benzodiazepines are readily used in many settings and have proven useful for their anxiolytic, amnesic and anti-convulsant properties (19). However, because of the risk of hypotension and secondary injury to the brain, care must be taken to ensure that the MAP does not drop significantly during induction. Respiratory depression must be avoided, bearing in mind that sedation may be prolonged from a build-up of metabolites (19). Benzodiazepines may also be useful for the purpose of ICP control, although a recent small retrospective cohort study demonstrated that bolus dosing of midazolam (together with fentanyl), was ineffectual in lowering ICP when used for episodic intracranial hypertension in children (20).
Etomidate is a useful sedative agent in children with severe TBI (21). It is especially relevant in the presence of hemodynamic instability, when the use of benzodiazepines is contraindicated. Etomidate, however, should be avoided among children at risk of adrenal suppression due to the known risk for relative adrenocortical insufficiency (22).
Propofol has demonstrated favorable effects in maintaining cerebral blood flow (CBF) among brain injured adult patients (23-25). However, propofol is not recommended in the acute resuscitation of a child with trauma because it can cause hypotension and decrease the CPP, particularly in children with multiple trauma or those with hypovolemia. Users should also monitor for propofol infusion syndrome, recognized by arrhythmias, metabolic acidosis, rhabdomyolysis, myoglobinuria, hyperlipidaemia, and fatty liver enlargement (26,27).
Ketamine is associated with improve outcomes in patients with TBI, contrary to prior beliefs that ketamine exacerbates raised ICP. MAP is either maintained or increased with the use of ketamine, as opposed to other sedative agents (28-30). In a study of 30 pediatric patients with intracranial hypertension, a single dose of ketamine prevented further increase in ICP during stressful interventions and reduced ICP among children with refractory intracranial hypertension (31). However, a systematic review of adults with TBI reported no significant difference in CPP, mortality, duration of intensive care unit (ICU) stay, or functional outcomes, with the use of ketamine (32).
No single sedative agent has proven superior in the adult severe TBI population, either in terms of mortality, functional outcomes, or in the treatment of raised ICP (33). As such, in the absence of known adrenal insufficiency, etomidate may be the sedative of choice. For unstable or hypotensive TBI patients, ketamine can be considered. If benzodiazepines are used, measures must be instituted to prevent and correct hypotension.
After optimizing sedation and establishing a secure airway, effective ventilation is essential to minimize secondary brain injury. Hyperventilation should not be performed routinely for children with TBI. Hyperventilation leads to cerebral vasoconstriction, reduced CBF and cerebral oxygenation, resulting in brain ischemia (34). Brain ischemia correlates proportionately with the extent of hyperventilation (35). In a retrospective cohort study, the more frequent the occurrences of severe hypocarbia among children with TBI, the greater the likelihood of mortality (36). In another retrospective cohort study, children with TBI and an admission PaCO2 between 36–45 mmHg were separately shown to have a better discharge outcome compared to those with admission hypocarbia (PaCO2 ≤35 mmHg) (37).
Current evidence comes mainly from observational studies. Prophylactic severe hyperventilation to PaCO2 <30 mmHg should be avoided in the initial resuscitation of a child with TBI (38). If hyperventilation is to be considered among patients with refractory intracranial hypertension, cerebral ischemia should be pre-empted and advanced neuromonitoring instituted (38).
Patients with TBI are known to be at risk for lung injury, which can be worsened by the use of high tidal volumes (39,40). This has led to the emergence of protective ventilation with new ventilation strategies (41). However, there is no clear evidence that the use of protective ventilation reduces mortality or duration of mechanical ventilation for TBI patients (42). The main ventilation goals in managing the child with TBI are still to avoid hypoxia and hypocarbia (43).
Hyperosmolar agents
The choice of hyperosmolar agent, dose and therapeutic threshold in management of TBI children with raised ICP vary between centers (44). While mannitol was used frequently in the past and is useful to reduce ICP (45), it has not been demonstrated to reduce mortality or improve functional outcomes (46). Mannitol use is associated with diuresis, hypovolemia and renal failure, raising concerns about routine use in raised ICP (47).
Hypertonic saline is being used increasingly and demonstrates effectiveness in rapid resolution of raised ICP (48,49). Hypertonic saline increases MAP (50), raises serum osmolality, and reduces cerebral edema. In the context of TBI, hypertonic saline increases the CPP and dampens spikes in ICP (51,52). Hypertonic saline also treats hypernatremia, which in patients with TBI can result from cerebral salt wasting, syndrome of inappropriate anti-diuretic hormone or sodium losses from cerebral spinal fluid drainage (38). Potential side effects, though rare, include central pontine myelinolysis, electrolyte abnormalities, rebound in ICP, and renal impairment (53).
Besides effectively reducing ICP (54), one randomized controlled trial in children reported shorter ICU stays, fewer interventions and shorter mechanical ventilation times with the use of hypertonic saline (55). In a retrospective study looking at children with TBI treated with hypertonic saline (56), there was a higher than expected survival rate, based on Injury Severity Score. Importantly, none of these patients developed adverse effects such as central pontine myelinolysis, pulmonary edema, renal impairment or rebound increase in ICP.
Hypertonic saline is recommended as part of standard therapy for children with raised ICP, in the management of TBI (38).
Therapeutic hypothermia
Therapeutic hypothermia was previously reported useful in the management of intracranial hypertension in TBI patients (57,58). Hutchison et al. (59) studied 225 children who were randomized either to hypothermia (32–33 °C for 48–72 hours) or normothermia, within 8 hours of injury. They found that the hypothermia group had an increased risk of hypotension and requirement for vasoactive agents especially in the rewarming period, and increased risk of mortality. However, there were concerns raised pertaining to the use of hyperventilation, and the speed of rewarming as part of the standard protocol.
In the phase III Cool Kids multicenter trial, patients younger than 18 years old were enrolled within 6 hours of injury and randomly allocated to either hypothermia (32–33 °C for 48–72 hours) or normothermia (60). There was no significant difference in mortality or global functional outcomes at 3 months. The study was terminated for futility (60). Subsequent meta-analyses suggest that hypothermia therapy is associated with increased risk of mortality when compared to normothermia (61,62). A recent Cochrane review included both adult and pediatric TBI studies with a cooling duration of at least 12 hours, but did not find evidence that that hypothermia therapy was associated with a favorable outcome (63).
Hypothermia also has an impact on drug metabolism. For example, therapeutic hypothermia slows down phenytoin elimination thereby increasing the exposure risk for drug toxicity (64).
Hence, normothermia should be maintained in the care of a child with TBI (62,65).
Glycemic control
Hyperglycemia is known to be associated with TBI. Multifarious causes increase glucose levels and also bring about transient insulin resistance. These include but are not limited to raised levels of stress hormones, activation of inflammatory cytokine pathways, hypothalamic-pituitary-adrenal axis and sympathetic autonomic nervous system, pituitary and/or hypothalamic dysfunction (66). Undergoing anesthesia, procedures and major surgery can also worsen hyperglycemia.
A recent retrospective study on pediatric TBI patients found that the presence of hyperglycemia at admission is associated with increased risk of mortality (67). Early hyperglycemia in TBI especially in the first twenty-four has been associated with adverse outcomes such as increased length of stay in the ICU, increased duration of invasive ventilation, higher morbidity and mortality (67-70).
Although glycemic control is crucial to minimize secondary complications due to neuron toxicity, the impact of strict glycemic control and the optimal target range in the setting of TBI has yet to be delineated based on current available literature. However, a few recent pediatric studies have shown poorer outcomes associated with a blood glucose of more than 11 mmol/L in the initial 12 to 24 hours after sustaining pediatric TBI (67,69,71).
Urgent neuroimaging
Children with TBI may have variable symptoms and signs that are age-dependent. The physician managing an acute brain injury needs to decide which child requires urgent neuroimaging. Although a computed tomography (CT) scan of the brain is able to identify and locate an intracranial bleed, it is also associated with radiation. Cancer incidence is reportedly significantly higher in children and adolescents who are exposed to CT scans compared to unexposed individuals (72).
Published clinical prediction tools (CHALICE, PECARN and CATCH) guide the physician when making neuroimaging decisions for children with head injury (73-75). Prospective observational studies comparing these prediction tools demonstrate that PECARN performed with the best sensitivity (76,77). Implementation of the PECARN prediction rule and using computerized clinical decision support to provide risks of clinically important TBI resulted in modest but variable decreases in CT use (78). A period of close monitoring potentially leads to more discretionary neuroimaging (79,80). The overall advantage of prompt diagnosis needs to be weighed against the disadvantage of radiation exposure complications.
ICP monitoring
ICP monitoring is an important component of multi-modal neurological monitoring for children with severe TBI. Although ICP monitoring is not prognostic of neurologic or overall outcome as compared to cranial imaging modalities, its use in guiding management has been regarded as standard of care (81-83). In actual practice however, there are varied practices in initiation and application of ICP monitoring (84). This is likely contributed by uncertainty regarding effectiveness of monitoring and insufficient data on survival benefit and impact on neurological outcomes (85-87).
There has yet to be high quality randomized studies focused on the effects of ICP monitoring especially in children with severe TBI (88). A recent national study in the United States found ICP monitoring to be associated with decreased mortality only for patients with initial Glasgow Coma Scale score of 3. However the same study showed ICP monitoring to also be associated with longer ICU stay, invasive ventilation, hospitalization, and inflated hospital bills (87). Some have suggested that a standardized ICP monitoring protocol may be associated with beneficial outcomes (84). Advancements in technology such as the development of a non-invasive transfontanelle monitor may encourage routine use of ICP monitoring when managing infants with moderate to severe TBI (89).
Prophylactic anti-epileptics
Post traumatic seizures (PTS) are often subtle (90) and are more likely to occur in younger children less than 24 months old (91), those with severe injury with GCS score lower than 8 (91) and non-accidental trauma (91-95). PTS are classified as early when they occur within 7 days of the head injury (96).
Although practice is variable (97,98), electroencephalographic (EEG) monitoring and the use of antiepileptic drugs (AED) are more common among children with known risk factors for PTS (99). The literature reporting on the efficacy of prophylactic AEDs has been controversial. A randomized controlled trial of 102 children showed that phenytoin did not reduce the PTS rate within 48 hours of head injury (100). However, this study had enrolment difficulties, significant lost to follow up, and an overall low seizure rate.
While randomized controlled trials remain the gold standard to generate evidence for TBI, it has been recognized that we currently lack sufficient understanding on many basic aspects of TBI to standardize the other areas of TBI management. This could contribute to negative or failed trials (101). Here we highlight a large observational study of 275 children with TBI, which found that the use of AEDs reduced the likelihood of early PTS (91). A randomized double-blind trial in adults also reported that phenytoin was associated with a significantly lower risk of early PTS (102).
Apart from phenytoin, levetiracetam has recently surfaced as an alternative for the prevention of PTS (103). However, in a prospective observational study, early PTS occurred frequently despite prophylaxis with levetiracetam. The authors cautioned against routine use before more studies conclude its effectiveness, particularly in young children and those suspected to have abusive head trauma (104). Thus, anti-epileptic prophylaxis in children with TBI cannot be routinely recommended until further conclusive evidence emerges. AEDs should still be considered if risk factors for PTS are present.
Conclusions
Many domains of pediatric TBI management are consensus driven and lack strong evidence (Table 1). Developing individualized strategies based on age, clinical presentation, presence of raised ICP, within the limitations of each healthcare resource setting, would enable emergency and critical care physicians to manage children with TBI more effectively (105).
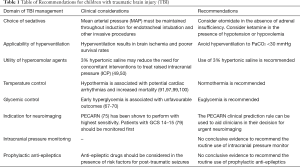
Full table
New treatment options are being explored (89,106). Priorities for future research (107-110) have been defined and will facilitate collaborations and larger studies (111). Comparative effectiveness research (101) has emerged as a powerful tool to harness heterogeneity in TBI management strategies across multinational centers and will likely be able to address some of these pressing clinical questions.
Acknowledgements
None.
Footnote
Conflicts of Interest: The authors have no conflicts of interest to declare.
References
- Keenan HT, Runyan DK, Nocera M. Longitudinal follow-up of families and young children with traumatic brain injury. Pediatrics 2006;117:1291-7. [Crossref] [PubMed]
- Ryan NP, van Bijnen L, Catroppa C, et al. Longitudinal outcome and recovery of social problems after pediatric traumatic brain injury (TBI): Contribution of brain insult and family environment. Int J Dev Neurosci 2016;49:23-30. [Crossref] [PubMed]
- Keenan HT, Bratton SL. Epidemiology and outcomes of pediatric traumatic brain injury. Dev Neurosci 2006;28:256-63. [Crossref] [PubMed]
- Rates of TBI-related Deaths by Age Group—United States, 2001-2010 [Internet]. Available online: https://www.cdc.gov/traumaticbraininjury/data/rates_deaths_byage.html
- Fulkerson DH, White IK, Rees JM, et al. Analysis of long-term (median 10.5 years) outcomes in children presenting with traumatic brain injury and an initial Glasgow Coma Scale score of 3 or 4. J Neurosurg Pediatr 2015;16:410-9. [Crossref] [PubMed]
- Tunthanathip T, Phuenpathom N. Impact of Road Traffic Injury to Pediatric Traumatic Brain Injury in Southern Thailand. J Neurosci Rural Pract 2017;8:601-8. [Crossref] [PubMed]
- Lichte P, Andruszkow H, Kappe M, et al. Increased in-hospital mortality following severe head injury in young children: results from a nationwide trauma registry. Eur J Med Res 2015;20:65. [Crossref] [PubMed]
- Shibahashi K, Sugiyama K, Okura Y, et al. Serious Concomitant Injuries in Pediatric Patients with Severe Traumatic Brain Injury. World Neurosurg 2017. [Epub ahead of print]. [PubMed]
- Wade SL, Zhang N, Yeates KO, et al. Social Environmental Moderators of Long-term Functional Outcomes of Early Childhood Brain Injury. JAMA Pediatr 2016;170:343-9. [Crossref] [PubMed]
- Dollman AK, Figaji AA, Schrieff-Elson LE. Academic and Behavioral Outcomes in School-Age South African Children Following Severe Traumatic Brain Injury. Front Neuroanat 2017;11:121. [Crossref] [PubMed]
- Gagner C, Landry-Roy C, Bernier A, et al. Behavioral consequences of mild traumatic brain injury in preschoolers. Psychol Med 2017.1-9. [Epub ahead of print]. [Crossref] [PubMed]
- Brown EA, Kenardy J, Chandler B, et al. Parent-Reported Health-Related Quality of Life in Children With Traumatic Brain Injury: A Prospective Study. J Pediatr Psychol 2016;41:244-55. [Crossref] [PubMed]
- Dewan MC, Mummareddy N, Wellons JC, et al. Epidemiology of Global Pediatric Traumatic Brain Injury: Qualitative Review. World Neurosurg 2016;91:497-509.e1. [Crossref] [PubMed]
- Schneier AJ, Shields BJ, Hostetler SG, et al. Incidence of pediatric traumatic brain injury and associated hospital resource utilization in the United States. Pediatrics 2006;118:483-92. [Crossref] [PubMed]
- Majdan M, Plancikova D, Brazinova A, et al. Epidemiology of traumatic brain injuries in Europe: a cross-sectional analysis. Lancet Public Health 2016;1:e76-e83. [Crossref] [PubMed]
- Cheng P, Yin P, Ning P, et al. Trends in traumatic brain injury mortality in China, 2006-2013: A population-based longitudinal study. PLoS Med 2017;14:e1002332. [Crossref] [PubMed]
- Chesnut RM, Marshall LF, Klauber MR, et al. The role of secondary brain injury in determining outcome from severe head injury. J Trauma 1993;34:216-22. [Crossref] [PubMed]
- Hardcastle N, Benzon HA, Vavilala MS. Update on the 2012 guidelines for the management of pediatric traumatic brain injury - information for the anesthesiologist. Paediatr Anaesth 2014;24:703-10. [Crossref] [PubMed]
- Flower O, Hellings S. Sedation in traumatic brain injury. Emerg Med Int 2012;2012:637171.
- Welch TP, Wallendorf MJ, Kharasch ED, et al. Fentanyl and Midazolam Are Ineffective in Reducing Episodic Intracranial Hypertension in Severe Pediatric Traumatic Brain Injury. Crit Care Med 2016;44:809-18. [PubMed]
- Bramwell KJ, Haizlip J, Pribble C, et al. The effect of etomidate on intracranial pressure and systemic blood pressure in pediatric patients with severe traumatic brain injury. Pediatr Emerg Care 2006;22:90-3. [Crossref] [PubMed]
- Malerba G, Romano-Girard F, Cravoisy A, et al. Risk factors of relative adrenocortical deficiency in intensive care patients needing mechanical ventilation. Intensive Care Med 2005;31:388-92. [Crossref] [PubMed]
- Farling PA, Johnston JR, Coppel DL. Propofol infusion for sedation of patients with head injury in intensive care. A preliminary report. Anaesthesia 1989;44:222-6. [Crossref] [PubMed]
- Pinaud M, Lelausque JN, Chetanneau A, et al. Effects of propofol on cerebral hemodynamics and metabolism in patients with brain trauma. Anesthesiology 1990;73:404-9. [Crossref] [PubMed]
- Chiu WT, Lin TJ, Lin JW, et al. Multicenter evaluation of propofol for head-injured patients in Taiwan. Surg Neurol 2006;66 Suppl 2:S37-42. [Crossref] [PubMed]
- Bray RJ. Propofol infusion syndrome in children. Paediatr Anaesth 1998;8:491-9. [Crossref] [PubMed]
- Junttila MJ, Gonzalez M, Lizotte E, et al. Induced Brugada-type electrocardiogram, a sign for imminent malignant arrhythmias. Circulation 2008;117:1890-3. [Crossref] [PubMed]
- Zeiler FA, Teitelbaum J, West M, et al. The ketamine effect on ICP in traumatic brain injury. Neurocrit Care 2014;21:163-73. [Crossref] [PubMed]
- Bourgoin A, Albanèse J, Léone M, et al. Effects of sufentanil or ketamine administered in target-controlled infusion on the cerebral hemodynamics of severely brain-injured patients. Crit Care Med 2005;33:1109-13. [Crossref] [PubMed]
- Filanovsky Y, Miller P, Kao J. Myth: Ketamine should not be used as an induction agent for intubation in patients with head injury. CJEM 2010;12:154-7. [Crossref] [PubMed]
- Bar-Joseph G, Guilburd Y, Tamir A, et al. Effectiveness of ketamine in decreasing intracranial pressure in children with intracranial hypertension: Clinical article. J Neurosurg Pediatr 2009;4:40-6. [Crossref] [PubMed]
- Cohen L, Athaide V, Wickham ME, et al. The effect of ketamine on intracranial and cerebral perfusion pressure and health outcomes: a systematic review. Ann Emerg Med 2015;65:43-51.e2. [Crossref] [PubMed]
- Roberts DJ, Hall RI, Kramer AH, et al. Sedation for critically ill adults with severe traumatic brain injury: a systematic review of randomized controlled trials. Crit Care Med 2011;39:2743-51. [Crossref] [PubMed]
- Curley G, Kavanagh BP, Laffey JG. Hypocapnia and the injured brain: more harm than benefit. Crit Care Med 2010;38:1348-59. [Crossref] [PubMed]
- Skippen P, Seear M, Poskitt K, et al. Effect of hyperventilation on regional cerebral blood flow in head-injured children. Crit Care Med 1997;25:1402-9. [Crossref] [PubMed]
- Curry R, Hollingworth W, Ellenbogen RG, et al. Incidence of hypo- and hypercarbia in severe traumatic brain injury before and after 2003 pediatric guidelines* Pediatr Crit Care Med 2008;9:141-6. [Crossref] [PubMed]
- Ramaiah VK, Sharma D, Ma L, et al. Admission oxygenation and ventilation parameters associated with discharge survival in severe pediatric traumatic brain injury. Childs Nerv Syst 2013;29:629-34. [Crossref] [PubMed]
- Kochanek PM, Carney N, Adelson PD, et al. Guidelines for the acute medical management of severe traumatic brain injury in infants, children, and adolescents--second edition. Pediatr Crit Care Med 2012;13 Suppl 1:S1-82.
- Kalsotra A, Zhao J, Anakk S, et al. Brain trauma leads to enhanced lung inflammation and injury: evidence for role of P4504Fs in resolution. J Cereb Blood Flow Metab 2007;27:963-74. [Crossref] [PubMed]
- Mascia L. Acute lung injury in patients with severe brain injury: a double hit model. Neurocrit Care 2009;11:417-26. [Crossref] [PubMed]
- Arora S, Singh PM, Trikha A. Ventilatory strategies in trauma patients. J Emerg Trauma Shock 2014;7:25-31. [Crossref] [PubMed]
- Luo XY, Hu YH, Cao XY, et al. Lung-protective Ventilation in Patients with Brain Injury: A Multicenter Cross-sectional Study and Questionnaire Survey in China. Chin Med J (Engl) 2016;129:1643-51. [Crossref] [PubMed]
- Agrawal S, Branco RG. Neuroprotective measures in children with traumatic brain injury. World J Crit Care Med 2016;5:36-46. [Crossref] [PubMed]
- Roumeliotis N, Dong C, Pettersen G, et al. Hyperosmolar therapy in pediatric traumatic brain injury: a retrospective study. Childs Nerv Syst 2016;32:2363-8. [Crossref] [PubMed]
- Mendelow AD, Teasdale GM, Russell T, et al. Effect of mannitol on cerebral blood flow and cerebral perfusion pressure in human head injury. J Neurosurg 1985;63:43-8. [Crossref] [PubMed]
- Misra UK, Kalita J, Ranjan P, et al. Mannitol in intracerebral hemorrhage: a randomized controlled study. J Neurol Sci 2005;234:41-5. [Crossref] [PubMed]
- Exo J, Smith C, Smith R, et al. Emergency treatment options for pediatric traumatic brain injury. Ped Health 2009;3:533-41. [Crossref] [PubMed]
- Bennett TD, Statler KD, Korgenski EK, et al. Osmolar therapy in pediatric traumatic brain injury* Crit Care Med 2012;40:208-15. [Crossref] [PubMed]
- Shein SL, Ferguson NM, Kochanek PM, et al. Effectiveness of Pharmacological Therapies for Intracranial Hypertension in Children With Severe Traumatic Brain Injury—Results From an Automated Data Collection System Time-Synched to Drug Administration Pediatr Crit Care Med 2016;17:236-45. [Crossref] [PubMed]
- Samant UB, Mack CD, Koepsell T, et al. Time of hypotension and discharge outcome in children with severe traumatic brain injury. J Neurotrauma 2008;25:495-502. [Crossref] [PubMed]
- Rallis D, Poulos P, Kazantzi M, et al. Effectiveness of 7.5% hypertonic saline in children with severe traumatic brain injury. J Crit Care 2017;38:52-6. [Crossref] [PubMed]
- Khanna S, Davis D, Peterson B, et al. Use of hypertonic saline in the treatment of severe refractory posttraumatic intracranial hypertension in pediatric traumatic brain injury. Crit Care Med 2000;28:1144-51. [Crossref] [PubMed]
- Qureshi AI, Suarez JI. Use of hypertonic saline solutions in treatment of cerebral edema and intracranial hypertension. Crit Care Med 2000;28:3301-13. [Crossref] [PubMed]
- Fisher B, Thomas D, Peterson B. Hypertonic saline lowers raised intracranial pressure in children after head trauma. J Neurosurg Anesthesiol 1992;4:4-10. [Crossref] [PubMed]
- Simma B, Burger R, Falk M, et al. A prospective, randomized, and controlled study of fluid management in children with severe head injury: lactated Ringer’s solution versus hypertonic saline. Crit Care Med 1998;26:1265-70. [Crossref] [PubMed]
- Peterson B, Khanna S, Fisher B, et al. Prolonged hypernatremia controls elevated intracranial pressure in head-injured pediatric patients. Crit Care Med 2000;28:1136-43. [Crossref] [PubMed]
- Li H, Lu G, Shi W, et al. Protective effect of moderate hypothermia on severe traumatic brain injury in children. J Neurotrauma 2009;26:1905-9. [Crossref] [PubMed]
- Biswas AK, Bruce DA, Sklar FH, et al. Treatment of acute traumatic brain injury in children with moderate hypothermia improves intracranial hypertension. Crit Care Med 2002;30:2742-51. [Crossref] [PubMed]
- Hutchison JS, Ward RE, Lacroix J, et al. Hypothermia Therapy after Traumatic Brain Injury in Children. N Engl J Med 2008;358:2447-56. [Crossref] [PubMed]
- Adelson PD, Wisniewski SR, Beca J, et al. Comparison of hypothermia and normothermia after severe traumatic brain injury in children (Cool Kids): a phase 3, randomised controlled trial. Lancet Neurol 2013;12:546-53. [Crossref] [PubMed]
- Hutchison JS, Guerguerian AM. Cooling of children with severe traumatic brain injury. Lancet Neurol 2013;12:527-9. [Crossref] [PubMed]
- Crompton EM, Lubomirova I, Cotlarciuc I, et al. Meta-Analysis of Therapeutic Hypothermia for Traumatic Brain Injury in Adult and Pediatric Patients. Crit Care Med 2017;45:575-83. [Crossref] [PubMed]
- Lewis SR, Evans DJ, Butler AR, et al. Hypothermia for traumatic brain injury. Cochrane Database Syst Rev 2017;9:CD001048. [PubMed]
- Empey PE, Velez de Mendizabal N, Bell MJ, et al. Therapeutic Hypothermia Decreases Phenytoin Elimination in Children with Traumatic Brain Injury* Crit Care Med 2013;41:2379-87. [Crossref] [PubMed]
- Beca J, McSharry B, Erickson S, et al. Hypothermia for Traumatic Brain Injury in Children-A Phase II Randomized Controlled Trial. Crit Care Med 2015;43:1458-66. [Crossref] [PubMed]
- Shi J, Dong B, Mao Y, et al. Review: Traumatic brain injury and hyperglycemia, a potentially modifiable risk factor. Oncotarget 2016;7:71052-61. [Crossref] [PubMed]
- Fu YQ, Chong SL, Lee JH, et al. The impact of early hyperglycaemia on children with traumatic brain injury. Brain Inj 2017;31:396-400. [Crossref] [PubMed]
- Smith RL, Lin JC, Adelson PD, et al. Relationship between hyperglycemia and outcome in children with severe traumatic brain injury. Pediatr Crit Care Med 2012;13:85-91. [Crossref] [PubMed]
- Chong SL, Harjanto S, Testoni D, et al. Early Hyperglycemia in Pediatric Traumatic Brain Injury Predicts for Mortality, Prolonged Duration of Mechanical Ventilation, and Intensive Care Stay. Int J Endocrinol 2015;2015:719476.
- Liu-DeRyke X, Collingridge DS, Orme J, et al. Clinical impact of early hyperglycemia during acute phase of traumatic brain injury. Neurocrit Care 2009;11:151-7. [Crossref] [PubMed]
- Elkon B, Cambrin JR, Hirshberg E, et al. Hyperglycemia: an independent risk factor for poor outcome in children with traumatic brain injury*. Pediatr Crit Care Med 2014;15:623-31. [Crossref] [PubMed]
- Mathews JD, Forsythe AV, Brady Z, et al. Cancer risk in 680,000 people exposed to computed tomography scans in childhood or adolescence: data linkage study of 11 million Australians. BMJ 2013;346:f2360. [Crossref] [PubMed]
- Dunning J, Daly JP, Lomas JP, et al. Derivation of the children’s head injury algorithm for the prediction of important clinical events decision rule for head injury in children. Arch Dis Child 2006;91:885-91. [Crossref] [PubMed]
- Kuppermann N, Holmes JF, Dayan PS, et al. Identification of children at very low risk of clinically-important brain injuries after head trauma: a prospective cohort study. Lancet 2009;374:1160-70. [Crossref] [PubMed]
- Osmond MH, Klassen TP, Wells GA, et al. CATCH: a clinical decision rule for the use of computed tomography in children with minor head injury. CMAJ 2010;182:341-8. [Crossref] [PubMed]
- Babl FE, Borland ML, Phillips N, et al. Accuracy of PECARN, CATCH, and CHALICE head injury decision rules in children: a prospective cohort study. Lancet 2017;389:2393-402. [Crossref] [PubMed]
- Easter JS, Bakes K, Dhaliwal J, et al. Comparison of PECARN, CATCH, and CHALICE rules for children with minor head injury: a prospective cohort study. Ann Emerg Med 2014;64:145-52, 152.e1-5.
- Dayan PS, Ballard DW, Tham E, et al. Use of Traumatic Brain Injury Prediction Rules With Clinical Decision Support. Pediatrics 2017;139:e20162709. [Crossref] [PubMed]
- Nigrovic LE, Schunk JE, Foerster A, et al. The effect of observation on cranial computed tomography utilization for children after blunt head trauma. Pediatrics 2011;127:1067-73. [Crossref] [PubMed]
- Schonfeld D, Fitz BM, Nigrovic LE. Effect of the duration of emergency department observation on computed tomography use in children with minor blunt head trauma. Ann Emerg Med 2013;62:597-603. [Crossref] [PubMed]
- Kukreti V, Mohseni-Bod H, Drake J. Management of raised intracranial pressure in children with traumatic brain injury. J Pediatr Neurosci 2014;9:207-15. [Crossref] [PubMed]
- Alali AS, Gomez D, Sathya C, et al. Intracranial pressure monitoring among children with severe traumatic brain injury. J Neurosurg Pediatr 2015.1-10. [Epub ahead of print]. [PubMed]
- Woischneck D, Kapapa T. The prognostic reliability of intracranial pressure monitoring and MRI data in severe traumatic brain injury. Magn Reson Imaging 2017;36:210-5. [Crossref] [PubMed]
- Bennett TD, Riva-Cambrin J, Keenan HT, et al. Variation in intracranial pressure monitoring and outcomes in pediatric traumatic brain injury. Arch Pediatr Adolesc Med 2012;166:641-7. [Crossref] [PubMed]
- Chesnut RM, Temkin N, Carney N, et al. A Trial of Intracranial-Pressure Monitoring in Traumatic Brain Injury. N Engl J Med 2012;367:2471-81. [Crossref] [PubMed]
- Bennett TD, DeWitt PE, Greene TH, et al. Functional Outcome After Intracranial Pressure Monitoring for Children With Severe Traumatic Brain Injury. JAMA Pediatr 2017;171:965-71. [Crossref] [PubMed]
- Alkhoury F, Kyriakides TC. Intracranial Pressure Monitoring in Children With Severe Traumatic Brain Injury: National Trauma Data Bank-Based Review of Outcomes. JAMA Surg 2014;149:544-8. [Crossref] [PubMed]
- Horvat CM, Kochanek PM. Big Data Not Yet Big Enough to Determine the Influence of Intracranial Pressure Monitoring on Outcome in Children With Severe Traumatic Brain Injury. JAMA Pediatr 2017;171:942-3. [Crossref] [PubMed]
- Behmanesh B, Bartels M, Gessler F, et al. Noninvasive Transfontanelle Monitoring of the Intracerebral Pressure in Comparison With an Invasive Intradural Intracranial Pressure Device: A Prospective Study. Oper Neurosurg (Hagerstown) 2017;13:609-13. [Crossref] [PubMed]
- Brain Trauma Foundation. American Association of Neurological Surgeons. Congress of Neurological Surgeons; et al. Guidelines for the management of severe traumatic brain injury. XIII. Antiseizure prophylaxis. J Neurotrauma 2007;24 Suppl 1:S83-6. [PubMed]
- Liesemer K, Bratton SL, Zebrack CM, et al. Early Post-Traumatic Seizures in Moderate to Severe Pediatric Traumatic Brain Injury: Rates, Risk Factors, and Clinical Features. J Neurotrauma 2011;28:755-62. [Crossref] [PubMed]
- Yoon SY, Choi YJ, Park SH, et al. Traumatic Brain Injury in Children under Age 24 Months : Analysis of Demographic Data, Risk Factors, and Outcomes of Post-traumatic Seizure. J Korean Neurosurg Soc 2017;60:584-90. [Crossref] [PubMed]
- Bansal S, Kebede T, Dean NP, et al. Predictors of Acute Symptomatic Seizures After Intracranial Hemorrhage in Infants* Pediatr Crit Care Med 2014;15:750-5. [Crossref] [PubMed]
- Ateş O, Öndül S, Önal Ç, et al. Post-traumatic early epilepsy in pediatric age group with emphasis on influential factors. Childs Nerv Syst 2006;22:279-84. [Crossref] [PubMed]
- Arango JI, Deibert CP, Brown D, et al. Posttraumatic seizures in children with severe traumatic brain injury. Childs Nerv Syst 2012;28:1925-9. [Crossref] [PubMed]
- Giza CC, Mink RB, Madikians A. Pediatric traumatic brain injury: not just little adults Curr Opin Crit Care 2007;13:143-52. [Crossref] [PubMed]
- Ostahowski PJ, Kannan N, Wainwright MS, et al. Variation in seizure prophylaxis in severe pediatric traumatic brain injury. J Neurosurg Pediatr 2016;18:499-506. [Crossref] [PubMed]
- Kurz JE, Poloyac SM, Abend NS, et al. Variation in Anticonvulsant Selection and Electroencephalographic Monitoring Following Severe Traumatic Brain Injury in Children—Understanding Resource Availability in Sites Participating in a Comparative Effectiveness Study Pediatr Crit Care Med 2016;17:649-57. [Crossref] [PubMed]
- Ruzas CM, DeWitt PE, Bennett KS, et al. EEG Monitoring and Antiepileptic Drugs in Children with Severe TBI. Neurocrit Care 2017;26:256-66. [Crossref] [PubMed]
- Young KD, Okada PJ, Sokolove PE, et al. A randomized, double-blinded, placebo-controlled trial of phenytoin for the prevention of early posttraumatic seizures in children with moderate to severe blunt head injury. Ann Emerg Med 2004;43:435-46. [Crossref] [PubMed]
- Approaches and Decisions in Acute Pediatric TBI Trial [Internet]. Available online: http://www.adapttrial.org/
- Temkin NR, Dikmen SS, Wilensky AJ, et al. A randomized, double-blind study of phenytoin for the prevention of post-traumatic seizures. N Engl J Med 1990;323:497-502. [Crossref] [PubMed]
- Klein P, Herr D, Pearl PL, et al. Results of Phase 2 Safety and Feasibility Study of Treatment With Levetiracetam for Prevention of Posttraumatic Epilepsy. Arch Neurol 2012;69:1290-5. [Crossref] [PubMed]
- Chung MG, O’Brien NF. Prevalence of Early Posttraumatic Seizures in Children With Moderate to Severe Traumatic Brain Injury Despite Levetiracetam Prophylaxis*. Pediatr Crit Care Med 2016;17:150-6. [Crossref] [PubMed]
- Scaife ER, Statler KD. Traumatic brain injury: preferred methods and targets for resuscitation Curr Opin Pediatr 2010;22:339-45. [Crossref] [PubMed]
- Clark RSB, Empey PE, Bayır H, et al. Phase I randomized clinical trial of N-acetylcysteine in combination with an adjuvant probenecid for treatment of severe traumatic brain injury in children. PLoS One 2017;12:e0180280. [Crossref] [PubMed]
- Adelson PD, Pineda J, Bell MJ, et al. Common Data Elements for Pediatric Traumatic Brain Injury: Recommendations from the Working Group on Demographics and Clinical Assessment. J Neurotrauma 2012;29:639-53. [Crossref] [PubMed]
- Berger RP, Beers SR, Papa L, et al. Common Data Elements for Pediatric Traumatic Brain Injury: Recommendations from the Biospecimens and Biomarkers Workgroup. J Neurotrauma 2012;29:672-7. [Crossref] [PubMed]
- Duhaime AC, Holshouser B, Hunter JV, et al. Common data elements for neuroimaging of traumatic brain injury: pediatric considerations. J Neurotrauma 2012;29:629-33. [Crossref] [PubMed]
- McCauley SR, Wilde EA, Anderson VA, et al. Recommendations for the Use of Common Outcome Measures in Pediatric Traumatic Brain Injury Research. J Neurotrauma 2012;29:678-705. [Crossref] [PubMed]
- Bell MJ, Kochanek PM. Pediatric Traumatic Brain Injury in 2012. Crit Care Clin 2013;29:223-38. [Crossref] [PubMed]
Cite this article as: Teng SS, Chong SL. Pediatric traumatic brain injury—a review of management strategies. J Emerg Crit Care Med 2018;2:18.