Management of severe chest wall trauma
Anatomy and physiology of chest trauma
The skin and soft tissue overlie the musculature of the bone-foundation creating the barrel-like structure of the chest wall. The bony thorax is comprised of the sternum, 12 ribs, the clavicles, the scapulae, and the vertebrae. Aiding in respiration and stabilizing the chest wall are the intercostal muscles and diaphragm, and the latissimus dorsi, serratus anterior and pectoralis major and minor muscles. This design protects the thoracoabdominal viscera and allows for efficient volumetric changes in the chest dimension. The thoracic “cage” moves in conjunction with the musculature of the chest wall changing dimension with each breath to alter the intrathoracic pressures with inspiration and expiration. The inspiratory and expiratory pressure gradients assist the ventilation of the lungs, which are mechanically in series with the chest wall.
The intimate association of the chest wall structure with the functional mechanics of the thorax underlies the pathophysiology of chest trauma. Injury to the chest can affect respiratory function in two capacities:
- Deformity of the chest wall may alter respiratory mechanics leading to hypercarbia, respiratory failure and respiratory acidosis;
- Increased shunt fraction (Qs/Qt) via pulmonary contusion, hemorrhage or edema from energy transfer or shear-type forces at the inertial interfaces causing hypoxemia.
More often chest wall trauma encompasses both physiologic insults, and can be compounded by resuscitation efforts, volume overload, and systemic inflammation. Furthermore, pneumothorax, hemothorax, tension physiology and cardiac tamponade compromise gas exchange and lead to circulatory dysfunction.
In the severely injured patient, damage control resuscitation with aggressive correction of acidosis, hypothermia, and coagulopathy is tantamount to patient treatment. Judicious fluid administration must be carefully delivered to avoid hypoperfusion while minimizing lung edema and blossoming of pulmonary contusions. Furthermore, up to 15% of polytraumas will require massive transfusion (>10 units of red blood cell transfusion) with potentially additive complications of transfusion-related acute lung injury (TRALI) and transfusion associated circulatory overload (TACO) seen in 15% and 8% of blood transfusions, respectively (1-4). Each of these physiologic insults adds to the morbidity and mortality of the patient’s injury.
Initial trauma bay management is in support of cardiopulmonary circuit per the airway, breathing, and circulation (ABC)’s of trauma management. Recognition of the need for ventilator support and the use of thoracostomy tubes to decompress hemorrhage and tension pneumothoraxes is essential to early management.
Airway: tracheobronchial injuries (TBIs)
Severe acceleration/deceleration injuries may be associated with TBI. Manifestations may vary from incidental mediastinal air to respiratory collapse. TBIs are typically found within 2 cm of the carina with a higher likelihood of occurrence on the right bronchus or distal trachea from shearing of the inertial interfaces.
Tracheal-bronchial disruptions should be suspected in the following circumstances:
- Mediastinal air in isolation (without associated pneumothorax or lung laceration, no evidence for an aerated tract from a soft tissue injury nor infra-diaphragmatic source);
- Respiratory distress made worse with positive pressure ventilation;
- Continued and sustained air leak on well-placed chest tube;
- Severe subcutaneous emphysema (35–85% of cases);
- Blood within the airway/hemoptysis (25%) (3,5).
Bronchoscopy is both diagnostic and therapeutic, often allowing passage of the endotracheal tube beyond the injury or into the unaffected bronchus to stabilize the airway. Definitive surgical repair is critical for large or symptomatic injuries. Strategies to support ventilation and oxygenation are discussed later
Breathing: disruption of the bony thorax and pulmonary injury
Disruption of the thoracic “cage”
Rib fractures are common in chest trauma and are present in upwards of 10–20% of trauma patients. Children and young adults have more compliant, cartilaginous chest wall structures and therefore may sustain major intrathoracic injury in the absence of rib fractures. Underlying lung injury with pulmonary hemorrhage and contusions are the prominent cause of respiratory failure in this age group (6,7).
Low energy fractures of the ribs disproportionately affect the elderly owing to a frail and brittle bone structures (6,7). Nonetheless, profound morbidity is observed in the aged from loss of efficient respiratory mechanics, pain-related chest wall splinting, and underlying lung injury. For each additional rib fracture in the elderly, mortality increases by 19% and the risk of pneumonia by 27% (8). These patients also have a higher incidence of chronic lung disease and cardiovascular disease that can worsen outcomes (8,9). An oxygen saturation <92% on room air, a tidal volume under 1.4 L and an incentive spirometry volume under 15 cc/kg are markers of pending respiratory compromise.
In any patient with rib fractures or chest wall injury, the main goal in management is to minimize the sequelae of impaired chest wall mechanics. Pain from fractures and muscular injury to the chest wall leads to splinting, decreased inspiratory capacity, and decreased sputum clearance. In the acute period, this is done by providing adequate analgesia to maintain the patient’s ability to cough, take adequate tidal volumes, and mobilize. Oral and intravenous opioid analgesia are commonly used for patients with simple rib fractures. These therapies can be used as an adjunct to centrally acting non-opioid analgesia and anti-inflammatory medications when not contraindicated. To avoid the systemic effects of opioid analgesia, other modalities such as intercostal nerve blocks, paravertebral blocks, and epidural analgesia have been used. Intercostal nerve blocks have shown benefit in patients with rib fractures and involve the injection of local anesthetic agents near the neurovascular bundle. The advantage to this is the avoidance of opioid analgesia and less risk of central nervous system depression but typically lasts only 8–12 hours and need to be repeated to provide adequate long-term analgesia. Paravertebral nerve blocks are another option that can provide pain control without systemic effects of opioid analgesia. A catheter is inserted into the paravertebral space on the ipsilateral side as the fractures, which is then infused first with dilute bupivacaine with epinephrine and then a continuous infusion with bupivacaine. This provides a viable option for patients who have multiple rib fractures that may not be amenable to intercostal nerve blocks, or those who are coagulopathic or have vertebral fractures that preclude placement of an epidural catheter (10,11).
Epidural anesthesia is widely used in the treatment of pain from rib fractures. Epidural anesthesia agents include a local anesthetic, such as bupivacaine, with or without an opioid, administered into the thoracic epidural space. Disadvantages include potential hypotension, injury to the spinal cord, epidural hematoma, urinary retention and infection (12).
In instances of more severe trauma, the presence of multiple rib fractures should clue the clinician into higher energy transfer to the body. Rib fractures are an independent predictor of other abdominal solid organ injury associated with an odds ratio of 3.5 increased risk of liver or splenic trauma (13). Severe chest trauma is predominately from vehicle crashes and pedestrian trauma. Fracture patterns may involve multiple or bilateral ribs with displacement or comminution. Radiographically, a flail chest is defined as 3 or more consecutive ribs, each broken in two locations. The diagnosis of a flail segment, however, is a clinical diagnosis, if the flail segment displays exam findings of paradoxical motion with the respiratory cycle.
Diagnosis
Chest wall injuries may be diagnosed with an expeditious and thorough physical exam.
Vital signs with evidence of tachycardia, hypotension, tachypnea, cyanosis, pallor, and poor oxygenation saturation are signs of shock and harbingers of severe physiologic disturbance. Inspection of the chest may reveal chest wall asymmetry, open wounds, subcutaneous emphysema and crepitus, rib step offs, and flail segments. Relying on auscultation and percussion may be unreliable amidst the noise of the trauma bay, however, flow of air and the presence of breath sounds bilaterally are important findings to note. The absence of breath sounds should prompt the clinician to evaluate the depth of the endotracheal tube (if present) to assure a tracheal, not main stem intubation. If breath sounds are absent with hemodynamic instability, an intervention to decompress the chest should be made without imaging. Other classic signs of cardiac trauma such a muffled heart sounds or Hamman’s sign are difficult to assess in the initial workup of the patient.
The extended focused assessment with sonography for trauma, or e-FAST, has been increasingly utilized as an extension of the physical exam. The exam is a quick, bedside test that uses ultrasonography of the inter-rib space to evaluate pleural apposition. The absence of pleural apposition diagnoses the presence of a pneumothorax and/or effusion. The extended views of the chest are utilized in conjunction with the classic FAST exam which evaluates the pericardium, the right upper and left upper quadrant recesses and the pelvis for free fluid. These ultrasonographical detect 92–100% of all pneumothoraces (14,15).
Chest radiographs are crucial to the diagnosis of chest trauma and should be obtained and interpreted before the patient is transported from the trauma bay. A methodical approach to the radiograph is necessary to evaluate the airway and bilateral hemithoraces, the cardio-mediastinal silhouette, the diaphragmatic contours. Bony disruption and subcutaneous emphysema are readily diagnosed on anteroposterior (AP) radiograph. The appropriate placement of the endotracheal tube and access lines must be confirmed and the presence of foreign bodies identified. The portable chest X-ray is easily obtained in the trauma bay and is the first-line diagnostic study, However, chest radiographs may miss non-displaced rib fractures, vertebral fractures, aortic trauma, and up to 20% of pneumothoraces.
In hemodynamically stable patients, chest CT offers higher resolution imaging and is far more sensitive in diagnosis of small anterior pneumothoraxes, lung contusions and lung lacerations, as well as bony injury of the sternum, scapula, ribs and spine. The increased diagnostic yield however, may only matter to clinical management in a few instances. CT remains is the gold standard in the evaluation of the cervico-thoracic spine. Additionally, in patients with a severe energetic mechanism or widened mediastinum, CT may help definitively diagnose aortic injury (6,7). Lastly, for stable patients with extensive chest wall injuries the use of 3D reconstruction is often helpful to operative planning for potential rib fixation.
Rib fixation
Operative reduction and fixation (ORIF) of the chest wall has been evolving as an additional method of chest wall stabilization. The revised Eastern Association for the Surgery of Trauma (EAST) guidelines, “conditionally recommend ORIF in adult patients with flail chest to decrease mortality, shorten duration of mechanical ventilation, incidence of pneumonia and need for tracheostomy” (level III evidence) (16). Those who are candidates for operative fixation generally have significant pain that is difficult to control, and respiratory impairment from the impact of the flail segment on the mechanics of the chest wall.
Over the years, many attempts have been made to fashion devices to stabilize the chest wall. These devices have evolved from simple silk suture fixation to measured plates in anatomical configuration and bracketed fixators. The simple idea behind these devices has been to stabilize the chest wall to improve pain and respiratory mechanics. Multiple randomized controlled trials have indicated decreased rates of pneumonia and shorter time on the ventilator when patients with rib fractures are treated with surgical fixation (16-30).
Two randomized controlled trial deserve mention. Tanaka et al. performed a prospective study of 37 patients matched by injury severity score (ISS), severity of pulmonary contusion and admission P/F ratio. The control group was managed with internal stabilization with positive end-expiratory pressure (PEEP), synchronous intermittent mandatory ventilation (SIMV) and pressure support. The results reached statistical significance favoring operative fixation with 8 days less of mechanical ventilation, 10 fewer intensive care unit (ICU) days and 50% reduction in pneumonia. Long-term outcomes for return to work also heavily favored operative stabilization (28). A more contemporary study by Marasco et al. randomized 46 patients to surgical stabilization with resorbable plates versus mechanical ventilation also significantly favored surgical stabilization, although less dramatically that the Tanaka study, with a 26% reduction in pneumonia and a 3-day decrease in ICU stay (27). Two recent a meta-analysis has evaluated surgical fixation. The first by Leinicke, included nine studies with 538 patients reported a significant decrease in primary outcome, which was duration of mechanical ventilation [pooled effect size (ES), −4.52 days; 95% confidence interval (CI), −5.54 to −3.50). For secondary outcomes which included ICU length of stay (ICULOS), hospital length of stay (HLOS), mortality, pneumonia, and tracheostomy, pooled analysis showed significantly decreased incidence in the operative fixation group; ICULOS (pooled ES, −3.40 days; 95% CI, −6.01, −0.79), HLOS (pooled ES, −3.82 days; 95% CI −7.12, −0.54), and decreased mortality [pooled relative risk (RR), 0.44; 95% CI, 0.28, 0.69], pneumonia (pooled RR, 0.45; 95% CI, 0.30, 0.69), and tracheostomy (pooled RR, 0.25; 95% CI, 0.13, 0.47) (16,18-20)].
The second meta-analysis by Kasotakis et al. was utilized for the basis of the EAST guidelines evaluated 22 studies for a total of 986 patients of which 334 underwent rib ORIF. This included both retrospective and three randomized controlled trials (including Tanaka and Marasco). The data had a wide variety of surgical techniques including struts, wires and plates; as well as, significant heterogeneity in the conservative management strategies of intubation, tracheostomy and fluid management. Only one study evaluated pain as an outcome and incorporated 37 patients. Surgical rib stabilization demonstrated consistent improvement in duration of mechanical ventilation, ICU and HLOS, pneumonia rates and need for tracheostomy (19). Although the available evidence is not without its limitations, there is randomized controlled prospective data supporting surgical fixation in appropriately selected patients (13,14,16,19-25,27,28) (see Table 1)
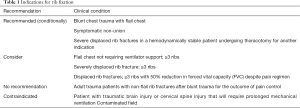
Full table
There have been several techniques described to stabilize the rib, and currently there is no evidence to support one method of fixation over another. Previously, wire cerclage was used to secure anterior metal plates to bridge the fracture. Given the propensity for fracture of the wire itself and subsequent dislodgement of the plate, uni- and bi-cortical screws have now replaced wire cerclage as fixation points for the anterior plate. Bi-cortical screws are generally sized for the thickness of the rib, and care is taken to avoid placing screws through the posterior cortex and into the pleura. Judet struts, which are placed without screw fixation and similar U-plates with locking screws provide similar fixation without the need for a long span of healthy rib to anchor the device, but carry a theoretical risk of intercostal nerve impingement from the inferior portion of the hardware locking around the rib. Fixation should aim to immobilize the fracture enough to allow pain relief and healing, but leave room for micromovement at the fracture site to promote osteoclast activity and callous formation. Fractures of ribs 3–10 are considered for repair due to the difficulty in reaching ribs 1 and 2, and minimal contribution of these and ribs 11 and 12 to respiratory mechanics. Attempts should be made to stabilize both fracture lines, however, if additional exposure is necessary it may be sufficient to stabilize one fracture line without impairment in pain control or pulmonary function (30). Posterior fractures within 2.5 cm of the transverse process should not be repaired due to inadequate bone length to secure a plate or strut. Furthermore, the generation muscular support and stability afforded in this region usually obviates the need for fixation close to the spine. Additionally, techniques have been described to fix anterior fractures to the costal cartilage or sternum but are currently off label uses of the available products (Figures 1,2).
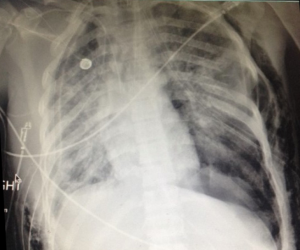
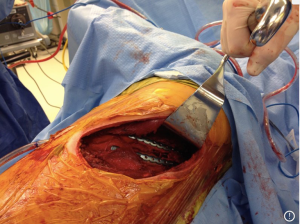
Ventilator modes/lung isolation
The treatment of the unstable chest injury has evolved significantly. Positive pressure ventilation physiologically acts as an internal “splint” against unstable chest wall. Termed “internal pneumatic stabilization” historic treatment of unstable chest segment was directed at early intubation and ventilatory support. However, this did not consider the patient’s baseline pulmonary function, and fell out of favor in the 1970s to 1980s after it was found to be associated with increased mortality due to ventilator associated complications (31,32). Mandatory mechanical ventilatory support solely for chest wall stabilization has been abandoned. The EAST guidelines state that “obligatory mechanical ventilation should be avoided (33). Increased use of noninvasive strategies involves intensive pulmonary toilet and involvement of respiratory therapy for volume expansion with incentive spirometry, noninvasive positive airway pressure, and cough-assist. High flow nasal cannula oxygen alone may be able to allow patients to avoid or delay mechanical ventilation. Two meta-analyses supported the use of noninvasive ventilation (NIV) in chest trauma. Roberts et al. performed a meta-analysis of 17 studies and Duggal et al. included nine studies on the use of to evaluate the safety and efficacy of NIV in chest trauma. Both included three randomized controlled trials. Although there was significant heterogeneity in terms of injury severity, level of hypoxemia and timing of intervention, NIV was safe with no increased morbidity and mortality. The rate of intubation, hospital and ICULOS in a patient population where 20% eventually required invasive ventilation (34,35). Chiumello et al. also evaluated ten studies with 386 patients meeting inclusion criteria. Overall arterial oxygen saturations were significantly higher with a significant reduction in intubation (36). Interestingly, no meta-analysis has shown a significant reduction in mortality with NIV (34-37).
If patients are requiring high flow oxygen or non-invasive ventilation close observation should be undertaken in the intensive care setting to monitor for acute decompensation or need for invasive ventilation. Attention to patients with concomitant intracranial injuries is required as these patients necessitate a higher arterial oxygen levels and hypoxemia should not be tolerated in this population.
The most severe forms of chest trauma warrant endotracheal intubation and mechanical ventilation for impending or manifest hypoxemic or hypercapnic respiratory failure. The insult of pulmonary contusion and subsequent alveolar-capillary leak can lead to poor lung compliance. The use of PEEP to recruit alveoli and decreased shunting is advised, and continuous positive airway pressure can be used to improve compliance. Several modes of ventilation have been studied, and no single mode has been proven more effective for patients with flail chest or pulmonary contusion (38,39). Management of ventilator modes should be left to provider comfort and familiarity.
Independent lung ventilation can be useful in the situation where a patient has a discrepant injury burden to each side of the chest resulting in a severely injured lung and a relatively normal contralateral lung. This allows lower tidal volumes and lung rest for the injured lung, which optimizing gas exchange with the healthy lung. There are few well-established indications for independent lung ventilation for trauma as the incidence is relatively rare. One such indication is the demonstration of paradoxical PEEP, which is hyperinflation of the healthy lung causing a fall in PaO2 and increased shunting to the injured lung. In such a situation, optimization of ventilation for the healthy lung could provide adequate gas exchange while allowing for recovery of the injured lung. Independent lung ventilation can be achieved through a single lumen endotracheal tube placed in the main-stem bronchus or by using a bronchial blocker.
Alternatively, dual-lumen endotracheal tubes are now widely available and can be used with two ventilators to provide separate ventilatory modes to each lung. If a dual-lumen endotracheal tube is being used, initial ventilator settings should correspond to equivalent ARDSNet protocols, adjusted for lung size. The clinician should keep in mind that high tidal volumes and airway pressure can themselves damage the already tenuous lung parenchyma. To decrease the incidence of ventilator associated lung injury, plateau pressures (Pplat) should be no greater than 30 cmH2O in the diseased lung. When managing the separate ventilators in independent lung ventilation, it is important to keep in mind the tidal volumes and airway pressures of each lung and to adjust accordingly to avoid barotrauma and optimize gas exchange. Transitioning from dual lumen to a single lumen endotracheal tube and conventional ventilation can be performed when lung compliance has allowed for equalization of tidal volume and gas exchange between the two lungs.
Extracorporeal membrane oxygenation (ECMO)
The first report of ECMO utilization in a trauma patient was put forth by Hill et al. (40) in 1972 with subsequent studies supporting the use of venovenous (V-V) ECMO in trauma patients. A relatively new mechanism for delivering ventilatory support, ECMO may be used in the setting of pulmonary failure when the lungs are so severely injured that mechanical ventilation has failed. Limited to case series reports, the reported survival ranges between 35–90% with large differences between published case series in trauma (2,41-49). V-V ECMO serves as temporary lung replacement in the setting of acute pulmonary failure with a reversible cause. ECMO effectively diverts blood flow to an extracorporeal membrane used for gas exchange, removing carbon dioxide outside the body, allowing mechanical ventilation to be delivered at minimal settings, or in some cases, not at all. This minimizes the chance for worsening damage to the lung parenchyma due to barotrauma, and allows a period of “lung rest” the lungs to recover while still providing gas exchange. ECMO is labor intensive and requires a highly skilled critical care team, and therefor is currently limited to specialized ECMO centers. Additionally, the diversion of blood flow to a mechanized environment is not without its own complications, and can be prone to thrombosis or the circuit. Circuits are typically run with systemic anticoagulation which may be contraindicated in trauma patients with concomitant injuries. In the acutely bleeding patient some centers will forego anticoagulation in patients undergoing V-V ECMO to minimize bleeding risk. Arlt et al. reported their experience with ten patients (mean ISS, 74±4) with percutaneous V-V and venoarterial (V-A) ECMO for pulmonary failure defined as (PaO2/FiO2 <100 mmHg) despite peep and advanced respiratory management. Patients were defined as meeting criteria for shock if they required >10 units transfusion or required vasopressor support despite fluid resuscitation. Overall survival was 60% with no thromboembolic events noted, nor decrease in ECMO function. Although limited in patient numbers, this small series gives equipoise to supporting appropriately selected patients who were previously contraindicated by bleeding risk, to an attempt at a heparin free circuit to support cardiopulmonary mechanics. Taking into consideration the often-fatal outcome of pulmonary failure in the setting of ISS score in the 1970’s any survival benefit from ECMO may be an improvement. Currently in trauma, ECMO is used as a bridge to allow time for pulmonary recovery in the setting of severe injury that is expected to resolve with time and lung rest to a potentially survivable outcome (2). The July 2016 International Summary ECLS Registry Report from the Extracorporeal Life Support Organization has shown for all patients with acute respiratory distress syndrome (ARDS) 58% survive to hospital discharge (45). Multi-institutional registry data is needed to fully expand the safety and utility of ECMO in trauma patients.
In summary, the management of chest wall trauma has evolved as we have made advances in surgical and critical care.
- A thorough initial diagnostic evaluation of severe chest trauma is essential to diagnosing major injury and advising therapeutic options;
- E-FAST may complement chest radiograph increase the detection of injury in the trauma bay;
- CT of the thorax is a useful adjunct to identifying vertebral injury, aortic injuries. Thoracic CT may clarify rib fracture anatomy and pulmonary injury in patient with significant chest trauma or in those who would benefit from surgical rib fixation;
- NIV may help bridge patients with some level of hypoxemia from chest injury, but should be carefully applied with close monitoring with clearly defined, patient specific thresholds for intubation should they deteriorate;
- Critically injured patients with hypoxemia and respiratory failure should be managed with mechanical ventilation;
- In patients with flail chest, fracture non-union, or >3 displaced rib fractures requiring mechanical ventilation or with 50% reduction in pulmonary function testing, rib fixation should be strongly considered;
- Finally, the use of ECMO in trauma recoverable patients that cannot be oxygenated and ventilated by traditional mechanical ventilation is in its infancy but is a promising treatment strategy to recuperate the lungs of the most severely injured patients.
Acknowledgements
None.
Footnote
Conflicts of Interest: The authors have no conflicts of interest to declare.
References
- Bernardin B, Troquet JM. Initial Management and Resuscitation of Severe Chest Trauma. Emerg Med Clin North Am 2012;30:377-400. [Crossref] [PubMed]
- Arlt M, Philipp A, Voelkel S. t al. Extracorporeal membrane oxygenation in severe trauma patients with bleeding shock. Resuscitation 2010;81:804-9. [Crossref] [PubMed]
- Benson AB, Austin GL, Mcfann KK, et al. Transfusion related acute lung injury in ICU patients with gastrointestinal bleeding. Intensive Care Med 2010;36:1710-7. [Crossref] [PubMed]
- Skeate RC, Eastlund T. Distinguishing between transfusion related acute lung injury and transfusion associated circulatory overload. Curr Opin Hematol 2007;14:682-7. [Crossref] [PubMed]
- Karmy-Jones R, Wood DE. Traumatic injury to the trachea and bronchus. Thorac Surg Clin 2007;17:35-46. [Crossref] [PubMed]
- Hauser CJ, Saillant NN, Livingston DH. Pulmonary Contusion and Flail Chest. In: Asensio JA, Trunkey DD. editors. Current Therapy of Trauma and Surgical Critical Care. 2nd edition. Philadelphia: Mosby-Elsevier, 2015:245-53.
- Livingston DH, Hauser C, Saillant NN, et al. Thoracic wall injuries: Ribs, sternal, and scapular fractures: Hemothoraces and pneumothoraces. In: Asensio JA, Trunkey DD. editors. Current Therapy of Trauma and Surgical Critical Care. 2nd edition. Philadelphia: Mosby-Elsevier, 2015:229-308.
- Barnea Y, Kashtan H, Skornick Y, et al. Isolated rib fractures in elderly patients: mortality and morbidity. Can J Surg 2002;45:43-6. [PubMed]
- Battle CE, Hutchings H, Evans PA. Risk factors that predict mortality in patients with blunt chest wall trauma: a systematic review and meta-analysis. Injury 2012;43:8-17. [Crossref] [PubMed]
- Karmakar MK, Chui PT, Joynt GM, et al. Thoracic paravertebral block for management of pain associated with multiple fractured ribs in patients with concomitant lumbar spinal trauma. Reg Anesth Pain Med 2001;26:169-73. [Crossref] [PubMed]
- Karmakar MK, Critchley LA, Ho AM, et al. Continuous thoracic paravertebral infusion of bupivacaine for pain management in patients with multiple fractured ribs. Chest 2003;123:424-31. [Crossref] [PubMed]
- Bulger EM, Edwards T, Klotz P, et al. Epidural analgesia improves outcome after multiple rib fractures. Surgery 2004;136:426-30. [Crossref] [PubMed]
- Subedi N, Yadav BN, Jha S. Liver and spleen injuries and associated rib fractures: an autopsy study. J Forensic Res 2014;5:240. [Crossref]
- Ball CG, Kirkpatrick AW, Feliciano DV. The occult pneumothorax: what have we learned? Can J Surg 2009;52:E173-9. [PubMed]
- Zanobetti M, Coppa A, Nazerian P, et al. Chest Abdominal-Focused Assessment Sonography for Trauma during the primary survey in the Emergency Department: the CA-FAST protocol. Eur J Trauma Emerg Surg 2015. [Epub ahead of print]. [Crossref] [PubMed]
- Cataneo AJ, Cataneo DC, de Oliveira FH, et al. Surgical versus nonsurgical interventions for flail chest. Cochrane Database Syst Rev 2015.CD009919. [PubMed]
- Bhatnagar A, Mayberry J, Nirula R. Rib fracture fixation for flail chest: What is the benefit? J Am Coll Surg 2012;215:201-5. [Crossref] [PubMed]
- Majercik S, Pieracci F. Chest Wall Trauma. Thorac Surg Clin 2017;27:113-21. [Crossref] [PubMed]
- Kasotakis G, Hasenboehler EA, Streib EW, et al. Operative fixation of rib fractures after blunt trauma: A practice management guideline from the Eastern Association for the Surgery of Trauma. J Trauma Acute Care Surg 2017;82:618-26. [Crossref] [PubMed]
- Leinicke JA, Elmore L, Freeman BD, et al. Operative management of rib fractures in the setting of flail chest: a systematic review and meta-analysis. Ann Surg 2013;258:914-21. [Crossref] [PubMed]
- De Moya M, Nirula R, Biffly W. Rib Fixation: Who, what when? Trauma Surg Acute Care Open 2017;2:e000059. [Crossref]
- Cohen EA. Treatment of the flail chest by towel clip traction. Am J Surg 1955;90:517-21. [Crossref] [PubMed]
- Granetzny A, Abd El-Aal M, Emam E, et al. Surgical versus conservative treatment of flail chest. Evaluation of the pulmonary status. Interact Cardiovasc Thorac Surg 2005;4:583-7. [Crossref] [PubMed]
- Pieracci FM, Majercik S, Ali-Osman F, et al. Consensus statement: Surgical stabilization of rib fractures rib fracture colloquium clinical practice guidelines. Injury 2017;48:307-21. [Crossref] [PubMed]
- Pieracci FM, Lin Y, Rodil M, et al. A prospective, controlled clinical evaluation of surgical stabilization of severe rib fractures. J Trauma Acute Care Surg 2016;80:187-94. [Crossref] [PubMed]
- Slobogean GP, MacPherson CA, Sun T, et al. Surgical fixation vs nonoperative management of flail chest: A meta-analysis. J Am Coll Surg 2013;216:302-11.e1. [Crossref] [PubMed]
- Marasco SF, Davies AR, Cooper J, et al. Prospective randomized controlled trial of operative rib fixation in traumatic flail chest. J Am Coll Surg 2013;216:924-32. [Crossref] [PubMed]
- Tanaka H, Vukioka T, Yamaguti Y, et al. Surgical stabilization of internal pneumatic stabilization? A prospective randomized study of the management of severe flail chest patients. J Trauma 2002;52:727-32. [Crossref] [PubMed]
- Senekjian L, Nirula R. Rib fracture fixation indications and outcomes. Crit Care Clin 2017;33:153-65. [Crossref] [PubMed]
- Nickerson TP, Thiels CA, Kim BD, et al. Outcomes of complete versus partial surgical stabilization of flail chest. World J Surg 2016;40:236-41. [Crossref] [PubMed]
- Richter T, Ragaller M. Ventilation in chest trauma. J Emerg Trauma Shock 2011;4:251-9. [Crossref] [PubMed]
- Ried M, Bein T, Phillip A, et al. Extracorporeal lung support in trauma patients with severe chest injury and acute lung failure: a 10-year institutional experience. Critical Care 2013;17:R110. [Crossref] [PubMed]
- Simon B, Ebert J, Bokhari F, et al. Management of pulmonary contusion and flail chest: an Eastern Association for the Surgery of Trauma practice management guideline. J Trauma Acute Care Surg 2012;73:S351-61. [Crossref] [PubMed]
- Roberts S, Skinner D, Biccardi B, et al. The role of non-invasive ventilation in blunt chest trauma: systematic review and meta-analysis. Eur J Trauma Emerg Surg 2014;40:553-9. [Crossref] [PubMed]
- Duggal A, Perez P, Golan E, et al. Safety and efficacy of noninvasive ventilation in patients with blunt chest trauma: a systematic review. Crit Care 2013;17:R142. [Crossref] [PubMed]
- Chiumello D, Coppola S, Froio S, et al. Noninvasive ventilation in chest trauma: systematic review and meta-analysis. Intensive Care Med 2013;39:1171-80. [Crossref] [PubMed]
- Hernandez G, Fernandez R, Lopez-Reina P, et al. Noninvasive ventilation reduces intubation in chest trauma-related hypoxemia: A randomized clinical trial. Chest 2010;137:74-80. [Crossref] [PubMed]
- Platz JJ, Fabricant L, Norotsky M, et al. Thoracic trauma; injuries, evaluation, and treatment. Surg Clin N Am 2017;97:783-99. [Crossref] [PubMed]
- Sharma OP, Oswanski MF, Jolly S, et al. Perils of rib fractures. The American Surgeon 2008;74:310-4. [PubMed]
- Hill JD, O'Brien TG, Murray JJ, et al. Prolonged extracorporeal oxygenation for acute post-traumatic respiratory failure (shock-lung syndrome). Use of the Bramson membrane lung. N Engl J Med 1972;286:629-34. [Crossref] [PubMed]
- Ahmad SB, Menaker J, Kufera J, et al. Extracorporeal membrane oxygenation after traumatic injury. J Trauma Acute Care Surg 2017;82:587-91. [Crossref] [PubMed]
- Gothner M, Buchwald D, Strauch JT, et al. The use of double lumen cannula for veno-venous ECMO in trauma patients with ARDS. Scand J Trauma Resusc Emerg Med 2015;23:30. [Crossref] [PubMed]
- Guirand DM, Okoye OT, Schmidt BS, et al. Venovenous Extracorporeal life support improves survival in adult trauma patients with acute hypoxemic respiratory failure; a multicenter retrospective cohort. J Trauma Acute Care Surg 2014;76:1275-81. [Crossref] [PubMed]
- Madershahian N, Wittwer T, Strauch J, et al. Application of ECMO in multitrauma patients with ARDS as rescue therapy. J Card Surg 2007;22:180-4. [Crossref] [PubMed]
- Peek GJ, Mugford M, Tiruvoipati R, et al. CESAR Trial Collaboration. Efficacy and economic assessment of conventional ventilatory support versus extracorporeal membrane oxygenation for severe adult respiratory failure (CESAR): a multicentre randomised controlled trial. Lancet 2009;374:1351-63. [Crossref] [PubMed]
- Ull C, Schildhauer TA, Strauch JT, et al. Outcome measures of extracorporeal life support (ECLS) in trauma patients versus patients without trauma: a 7-year single-center retrospective cohort study. J Artif Organs 2017;20:117-24. [Crossref] [PubMed]
- Kim HS. Extracorporeal membrane oxygenation support in trauma versus nontrauma patients with on infectious acute respiratory failure. Artif Organs 2017;41:431-39. [Crossref] [PubMed]
- Wu SC, Chen WT, Lin HH, et al. Use of extracorporeal membrane oxygenation in severe traumatic lung injury with respiratory failure. Am J Emerg Med 2015;33:658-62. [Crossref] [PubMed]
- Zonies D, Merkel M. Advanced extracorporeal therapy in trauma. Curr Opin Crit Care 2016;22:578-83. [Crossref] [PubMed]
Cite this article as: Saillant NN, Sein V. Management of severe chest wall trauma. J Emerg Crit Care Med 2018;2:41.