Multidrug resistant bacteria in critically ill patients: a step further antibiotic therapy
Multidrug resistant infections in intensive care unit (ICU): numbers and facts
Since the discovery of penicillin in 1928, antibiotics transformed the management of bacterial infections and saved millions of lives. Many decades later, a rising spread of antibiotic-resistant microorganisms led bacterial infections to become a threat again. The drug-resistance phenomenon already imposes a very heavy burden on healthcare, with 23,000 and 25,000 estimated annual deaths respectively in United States and in Europe. Moreover, some further studies prognosticate worrying trends, with an expected rising impact on global health through years, leading to more than 10 million annual deaths worldwide in 2050 (1-3).
ICUs are often considered the epicentre of development, amplification and dissemination of drug-resistant microorganisms (4,5). Critically ill patients are particularly prone to infections because of exposure to multiple invasive procedures compromising the anatomical barriers’ defences, impairment of protective mechanisms such as cough reflex or acid gastric ambient by sedative drugs or stress-ulcer prophylaxis and the frequent impairment of the immune response induced by trauma, surgery and sepsis (6,7). Furthermore, the use of broad-spectrum antibiotics, that is closely related to development and spread of drug-resistant microorganisms, is really frequent in ICU clinical practice, with studies reporting a 30% to 60% rate of inappropriate or incorrect antibiotic prescriptions (8-10). For these reasons nosocomial infections, often caused by multidrug resistant bacteria (MDR) micro-organisms, are more common in ICUs than in other departments (11).
A large-number of observational studies identified numerous independent risk factors for occurrence of infections by MDR bacteria among ICU patients: central venous access, pulmonary artery catheterization, stress ulcer prophylaxis, urinary catheterization, mechanical ventilation, trauma, ICU length of stay and, mostly, a previous history of infection or colonization by MDR micro-organisms (11,12). The prevalence of infections sustained by MDR bacteria in ICU patients varies in the different regions of the world. In North America, a study on critically ill patients with pneumonia (DEFINE study) reported a 14.1% rate of MDR infections, while a large study on nosocomial bloodstream infections conducted in 24 ICUs distributed worldwide (EUROBACT study) showed on average a 47.8% MDR rate, including 20.5% and 0.5% of isolated microorganisms with extensively drug-resistant (XDR) and pan-drug-resistant (PDR) patterns, respectively, with a consistent variability among different participating countries ranging from 8% (Australia) to more than 75–80% (Turkey, Greece, Croatia, Serbia) (12,13).
Over the past years, as a consequence of the progressive lack of antibiotic active against resistant gram-negative microorganisms, gram positive MDR pathogens have been overtaken by gram negative strain infections (14). Among gram negative bacteria, the most frequent MDR micro-organisms isolated in critically ill patients are extended-spectrum beta-lactamases producers (ESBL) Enterobacteriaceae and MDR Pseudomonas aeruginosa (PA), Acinetobacter spp. and Stenotrophomonas maltophilia. Vancomycin-resistant enterococci and methicillin-resistant Staphylococcus aureus are the most common gram positive identified, even if their incidence is currently decreasing (14,15).
The impact of antibiotic resistance on patient’s outcome is still a debated topic: even though many studies reported a larger proportion of infections by MDR bacteria in non-survivors compared to survivors, it is still unclear if this is caused by difficulties in initial antibiotic therapy and/or to differences in microorganisms virulence and/or to differences in patients characteristics, particularly in terms of immune response (16). Indeed, MDR susceptibility pattern has been shown to be strongly associated to delay in appropriate antimicrobial treatment, that is one of the major factors influencing survival in patients with sepsis and septic shock (17,18). In fact, broad-spectrum antibiotic therapy within 1 hour after sepsis diagnosis is strongly recommended in the recently proposed Surviving Sepsis Campaign 1 h bundle (19). However, many authors and scientific societies expressed concerns on this recommendation that appears to rough and may encourage an excessive and harmful use of broad-spectrum agents when a more tailored approach might be advisable (20).
Antibiotic therapy: possible effective strategies
As described above, the increased incidence of MDR bacteria in critically ill patients is the consequence of inappropriate antibiotic therapy and large consumption of broad-spectrum antibiotics (21). Institution of an antimicrobial stewardship program (AMS) seems to be an effective strategy and is strongly recommended in ICUs with high prevalence of MDR bacteria with the aim of fighting drug-resistances, improving patient outcomes and reducing health care costs (21). Key issues of AMS programs are halting antibiotics in patients without infection and selection of the appropriate drug for empirical therapy that, as general rules, depends on clinical conditions of the patient, source of infection, local antimicrobial susceptibility patterns, patient microbiological history and previous therapy, avoiding to repeat the same antibiotics (22,23). Moreover, use of adequate dosages, early de-escalation of empirical broad spectrum antibiotics focusing the treatment on the isolated microorganism, switching to mono-therapy whenever possible and short course of therapy are also fundamental elements of AMS in critically ill patients (10).
As well known, nowadays the treatments effective against MDR bacteria are limited or missing for specific PDR strains. Due to their safety profile and broad efficacy against many microorganisms, beta-lactam antibiotics have been considered the first line therapy since many decades. Unfortunately, bacterial production of β lactamase enzymes increased worldwide making beta-lactams unsuitable as first line therapy for nosocomial infections in many regions of the world. As consequence, in the last years the use of carbapenems as first-line empiric therapy in critically ill patients grew enormously leading to a substantial increase in incidence of carbapenem resistant bacteria, by different mechanisms of resistance (24).
In several European regions, Klebsiella pneumoniae producing Carbapenemase (KPC-KP) is one of the most common MDR gram-negative pathogens in critically ill patients. Despite of the lack of high-quality studies, many observational experiences showed that combined therapy is more effective than mono-therapy in patients with severe infections whereas no differences were observed in patients with less severe infections (25-28). Various antibiotics with different combination strategies have been proposed for the KPC-KP treatment including colistin, aminoglycosides, tigecycline, fosfomycin, carbapenems at high dosages and, in the last months, ceftazidime/avibactam (29-31). In case of pneumonia, the combination of intravenous and nebulized antibiotics has also been used with success. Several molecules, as for instance meropenem/vaborbactam, imipenem/relebactam, plazomicin and cefiderocol, active against the different mechanisms responsible for carbapenem resistance are under evaluation and will be available in the near future (32).
Acinetobacter baumannii (AB) is also a frequent cause of nosocomial acquired infection in critically ill patients (33). In fact, it is the third microorganisms responsible for ventilator acquired pneumonia in European ICUs, after S. Aureus and P. aeruginosa (34-36). Although carbapenems are usually considered the first line agents for the management of severe infections by AB (exception for ertapenem, that is no active), their use is becoming limited in many areas because of the increasing resistance (37). In this case, it is reasonable to use alternative strategies including usually sulbactam, tigecycline and colistin, that ought to be preserved for strains resistant to all β-lactams, fluoroquinolones and tigecycline (38-40). The combination of carbapenems, sulbactam and colistin, or colistin and rifampicin, or colistin and anti-gram-positive agents have been also suggested and frequently used even though the clear evidences of effectiveness are lacking.
PA represents one of the most common cause of health-care-associated infection and it is responsible for severe bloodstream, respiratory, urinary tract and soft tissue infections in ICU patients (41,42). In the last years, as for the other gram-negative bacteria, it has been evidenced an important increasing in infections caused by MDR-PA, due to the acquisition of resistance genes from mobile genetic components like transposons and plasmids, or to the variety of expression and function of encoded mechanisms into chromosomes. For many authors the best treatment for MDR-PA infection remains a dilemma between the mono and the combination therapy (43). However, the last option is generally considered more prudent and rational in critically ill patients, in order to extend the spectrum of activity (44). At present, the empirical treatment against a suspected MDR-PA infection, according to the site of infection, is represented by the novel β-lactam and β-lactamase inhibitor combinations (ceftolozane/tazobactam and ceftazidime/avibactam), or by antipseudomonal β-lactam (piperacillin/tazobactam, cefepime, ceftazidime or carbapenem) plus an additional agent such as colistin, fosfomycin, aminoglycoside or quinolones (45). The combination ceftolozane/tazobactam, a new semi-synthetic cephalosporin and a well-established β lactamase inhibitor, is indicated for complicated intra-abdominal or urinary tract infections, while it is still under evaluation for other clinical uses, like pneumoniae.
Immunosuppression in sepsis and relationship with MDR infections
During sepsis, complex immune reactions take place in the host that desperately try to protect the organism from external (pathogens) or internal (self-molecules that start inflammation) insults, and trigger a variety of mechanisms that change with time, and which include the production and utilization of pro- and anti-inflammatory molecules (46). Interestingly, these two classes of molecules, which have opposite effects, are often concomitantly secreted in high amounts (47). As a result, most patients with sepsis rapidly display signs of profound immune activation as well as of immunosuppression (48). This results in a status of immunological paralysis whose resolution is crucial for the life of the patient.
Patients who experience a septic shock caused by MDR microorganisms are however a particular population, which is characterized by a very high mortality risk (49). To understand the reasons of this risk, we have to analyze the following points. On the one side, the previous exposure to an initial inappropriate antibiotic therapy that is not able to control the infection but that can affect the host’s defences could be responsible for altering the immune functions of the host (50). Indeed, an empiric antibiotic treatment can have devastating ecologic effects on the microenvironment, as it can promote a superinfection sustained by resistant bacteria or fungi. Noteworthy, important antigens of the pathogen can become cryptic, and/or specific clones that recognize such antigens can undergo inactivation, anergy or even apoptosis after a long chronic stimulation.
On the other side, the continuous activation of an immune system that typically has been fighting with a pathogen for several weeks, if not several months, also plays a main role. In this perspective, a chronic immune activation affects not only the cells that are supposed to recognize a given antigen, but also creates a microenvironment in which cells of the innate immunity (monocytes, neutrophils, among others), as well as those of the cognitive part (specific T and B cells), receive a variety of stimuli that do not help in performing their activities (51).
Finally, it must be considered that most patients with sepsis have an advanced age. The effects of aging on the immune system are well studied, and it is known that either specific or non-specific responses are involved. Moreover, it has been reported that old individuals display the phenomenon of inflammaging, in which a chronic, subclinical inflammatory status drives all the immune responses, and causes a low quality and often low quantity control of the infection or of autoimmune reactions (52,53).
As a result, clinicians have to cope with a very dangerous and potentially fatal association among a sepsis caused by MDR infections, an aged and chronically stimulated immune system, and the immune-paralysis typical of sepsis (54). Assuming that an MDR pathogen is not sensitive to antibiotics, the only possibility for the survival of the host is to benefit of an efficient immune response, whose modulation is actually the Holy Grail of the treatment of sepsis.
The strategy to block the immune activation and potent inflammation that is typical of early sepsis has given, at present, discouraging results as indicated by the fact that in more than 30 trials of diverse anti-cytokine or anti-inflammatory drugs no benefit was shown, or, in some cases, a reduced survival rate was reported (55). One of the possible reasons is that the negative modulation of inflammation showed detrimental effects because molecules produced during inflammation are crucial for the control of the pathogen. However, only recently a therapeutic approach has been described which is based either on the trigger of the residual immune resources, or on the inhibition of inhibitory molecules, the so-called “check-point inhibitors”. The therapeutic benefit of interleukin-7 (IL-7, a cytokine that triggers the production of new lymphocytes from the thymus and can maintain peripheral T cell homeostasis), or of granulocyte macrophage colony-stimulating factor (GM-CSF, a strong stimulator of innate immune response) are nowadays under evaluation by specific clinical trials (56). The last approach, which is successfully used in several types of cancer, including metastatic melanoma, is based upon antibodies against check point inhibitors such as programmed cell death protein-1 (PD1, or CD279) or against is ligand, the programmed cell death ligand 1 (PDL1, or CD274) (57-59).
Adjunctive therapies in MDR infections
Early identification, appropriate antibiotic therapy and prompt fluid resuscitation in patients with hypotension are the cornerstone for treatment of sepsis due to MDR infections. Nevertheless, given the increasing difficulties caused by the antibiotic resistance and the high prevalence of immune-suppression in patients affected by MDR infections, the use of specific adjunctive therapies seems to be reasonable and may have beneficial effects in this patients’ population.
Extracorporeal blood purification techniques
In sepsis, the high levels of circulating inflammatory mediators contribute to development of organs dysfunction and to the imbalance of immune response and, thus, different extracorporeal blood purification techniques have been proposed to remove these mediators. The use of high-volume hemofiltration in septic patients did not show any beneficial effects and similar result have been obtained, with cascade hemofiltration (60-62). Hemoperfusion with polymyxin-B cartridge for removal of endotoxins seemed to be effective in improving patients’ outcome but the recent negative results of the EUPHRATES trial poses again questions on its efficacy (63-65).
Similarly, plasma exchange appeared to be effective in the removal of inflammatory mediators and the association between plasma filtration and adsorption could be potentially even more effective. Unfortunately, a recent randomized control trial in patients with septic shock did not show significant benefits (66).
Pharmacological approaches
As described above, different molecules have been tested to evaluate the ability to improve host immune response and eventually to exert a synergic effect with antibiotic therapy. Initially, GM-CSF and interferon-γ (INF-γ) have been proposed and studied because of their effects on antigen presenting cells. A randomized trial in septic patients evaluating the effects of GM-CSF administration guided by mHLA-DR expression noticed a prompt restoring of immune-response, a reduced need of mechanical ventilation and a shorter ICU and hospital length of stay in treated patients (67). Furthermore, a meta-analysis of clinical trials in septic shock showed a more efficiency in infection clearance but no improvement in 28-day mortality in patients treated by GM-CSF (68). The use of INF-γ has been tested in case of trauma and burns with contrasting results. Noteworthy, even in these trials the use of HLA-DR expression on monocytes was used to identify patients that could benefit the most from this adjunctive therapy (69). The PD-1/PD-L pathway, normally acting during the immune response to limit an excessive lymphocytes activation, is highly up-regulated during sepsis and septic shock leading to inhibition of immune cells, anergy and promoting apoptosis (70,71). Murine models of sepsis have been used to demonstrate that block of this pathway is responsible for an improvement in survival moreover an in vitro study on lymphocytes from septic patients treated with anti-PD-1 or anti-PD-1L antibodies showed a decrease in apoptosis rate and a higher production of IL-2 and INF-γ (72-74). These observations suggest the use of PD-1 expression on T cells and/or PD-L expression on antigen presenting cells as a sound biomarker for immune dysfunction and, as mentioned above, the potential use of anti-PD-1 and anti-PD-L1 antibody in patients as an adjunctive therapy in patients with sepsis. Other pathways such as TIM-3, LAG-3 and CTLA-4, BTLA have been studied and proposed to monitor and direct immune- therapies in sepsis and septic shock, but so far clinical trials are lacking (75). Potential benefits of recombinant interleukins in sepsis and septic shock have been demonstrated in animals. In different murine models of sepsis, IL-7 administration is able to restore depleted T cells, induce proliferation and INF-γ secretion allowing an improvement in survival. A recent small clinical randomized trial (IRIS-7) reported that recombinant-IL-7 in patients with septic shock and severe lymphopenia is well tolerated and induce a 3- to 4-fold increase in absolute lymphocyte counts and in circulating CD4+ and CD8+ T cells, making it a promising therapy in these patients at high risk for mortality. Finally, the administration of intravenous immunoglobulins (Ig) has been also proposed and used since many years for compensate the reduction of circulating IgG and IgM observed in the first days of septic shock and for modulating the immune response (76,77). Interesting in this filed, two recently published experiences in Italy and Greece reported a beneficial effect with the early use of IgM enriched immunoglobulin preparations in patients with sepsis and septic shock sustained by MDR bacteria (49,78).
Immunization against MDR pathogens
Recently murine models have been also used to test the efficacy of immunization against infections caused by MDR pathogens. The progressive emergence of MDR-AB as important opportunistic pathogen characterized by limited treatment options induced different groups to focus their attention on the development of a vaccine to prevent infection. Ainsworth et al. showed that the use of an attenuated strain of MDR AB, obtained deleting the thioredoxin gene from a clinical isolate, to vaccinate mice is able to protect from a lethal form of infection inducing Ig production and resulting in a reduced pathology and organ burden compared to non-vaccinated mice (79). Also the use of specific outer membrane protein A obtained by AB has been used to vaccinate mice and showed an improvement in survival after MDR-AB infection compared to non-immunized mice (80). Other MDR pathogens, such as Mycobacterium tuberculosis and PA, are under study and antigen candidates have been identified as potential protective vaccines (81,82).
Conclusions
Sepsis continues to be the most important cause of mortality in ICU patients, particularly when infection is caused by difficult to manage infections as those sustained by MDR-PDR-XDR micro-organisms. Appropriate and timely antibiotic therapy using combination strategies or novel molecules is the cornerstone for treatment of complex infections. However, even in case of appropriate antibiotic strategies, the mortality rate of patients with infections by MDR agents remains higher than that observed in patients with sepsis by no-MDR bacteria, especially in patients infected by opportunistic PDR-XDR bacteria or fungi. In the last years, it has become clear that persistent immune dysfunction, a frequent occurrence in critically ill patients, plays a pivotal role in acquisition of secondary/breakthrough infections and, thus, special attention ought to be dedicated, together with the best antibiotic strategy and standard of care, to identification and management of this dysfunction in ICU patients with difficult infections (Table 1). To this aim, different promising strategies have been considered and are under evaluation by appropriate trials with the hope to finally win the fight against infections by MDR bacteria in the near future.
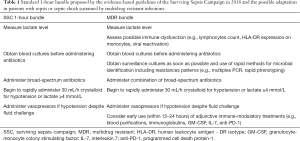
Full table
Acknowledgements
None.
Footnote
Conflicts of Interest: Massimo Girardis participated to advisory board and served as speaker for Accelix, Biomerieux, Biotest, MSD and Pfzier. The other authors have no conflicts of interest to declare.
References
- The review on antimicrobial resistance team chaired by O'Neil J. Antimicrobial Resistance: Tackling a Crisis for the Health and Wealth of Nations. Review on Antimicrobial Resistance 2014. Available online: https://amr-review.org/sites/default/files/AMR%20Review%20Paper%20-%20Tackling%20a%20crisis%20for%20the%20health%20and%20wealth%20of%20nations_1.pdf
- CDC. Antibiotic resistance threats in the United States, 2013. Available online: https://www.cdc.gov/drugresistance/pdf/ar-threats-2013-508.pdf
- The ECDC/EMEA joint working group. The bacterial challenge, time to react: a call to narrow the gap between multidrug-resistant bacteria in the EU and the development of new antibacterial agents. ECDC/ EMEA joint technical report. Stockholm: ECDC; 2009. Available online: https://ecdc.europa.eu/sites/portal/files/media/en/publications/Publications/0909_TER_The_Bacterial_Challenge_Time_to_React.pdf
- Kuhlen R. 25 Years of Progress and Innovation in Intensive Care Medicine. 1 edition. Berlin: Mwv, 2007.
- Brusselaers N, Vogelaers D, Blot S. The rising problem of antimicrobial resistance in the intensive care unit. Ann Intensive Care 2011;1:47. [Crossref] [PubMed]
- Hotchkiss RS, Monneret G, Payen D. Immunosuppression in sepsis: a novel understanding of the disorder and a new therapeutic approach. Lancet Infect Dis 2013;13:260-8. [Crossref] [PubMed]
- Conway Morris A, Datta D, Shankar-Hari M, et al. Cell-surface signatures of immune dysfunction risk-stratify critically ill patients: INFECT study. Intensive Care Med 2018;44:627-35. [Crossref] [PubMed]
- McGowan JE. Antimicrobial resistance in hospital organisms and its relation to antibiotic use. Rev Infect Dis 1983;5:1033-48. [Crossref] [PubMed]
- Marr JJ, Moffet HL, Kunin CM. Guidelines for improving the use of antimicrobial agents in hospitals: a statement by the Infectious Diseases Society of America. J Infect Dis 1988;157:869-76. [Crossref] [PubMed]
- Luyt CE, Bréchot N, Trouillet JL, et al. Antibiotic stewardship in the intensive care unit. Crit Care 2014;18:480. [Crossref] [PubMed]
- Vincent JL, Bihari DJ, Suter PM, et al. The prevalence of nosocomial infection in intensive care units in Europe. Results of the European Prevalence of Infection in Intensive Care (EPIC) Study. EPIC International Advisory Committee. JAMA 1995;274:639-44. [Crossref] [PubMed]
- Lat I, Daley MJ, Shewale A, et al. A multicenter, prospective, observational study to determine predictive factors for multidrug resistant pneumonia in critically ill adults: The DEFINE study. Pharmacotherapy 2018. [Epub ahead of print]. [Crossref] [PubMed]
- Tabah A, Koulenti D, Laupland K, et al. Characteristics and determinants of outcome of hospital-acquired bloodstream infections in intensive care units: the EUROBACT International Cohort Study. Intensive Care Med 2012;38:1930-45. [Crossref] [PubMed]
- Jones RN. Resistance patterns among nosocomial pathogens: trends over the past few years. Chest 2001;119:397S-404S. [Crossref] [PubMed]
- Boucher HW, Talbot GH, Bradley JS, et al. Bad Bugs, No Drugs: No ESKAPE! An Update from the Infectious Diseases Society of America. Clin Infect Dis 2009;48:1-12. [Crossref] [PubMed]
- Figueiredo Costa S. Impact of antimicrobial resistance on the treatment and outcome of patients with sepsis. Shock 2008;30 Suppl 1:23-9. [Crossref] [PubMed]
- Zilberberg MD, Nathanson BH, Sulham K, et al. Multidrug resistance, inappropriate empiric therapy, and hospital mortality in Acinetobacter baumannii pneumonia and sepsis. Crit Care 2016;20:221. [Crossref] [PubMed]
- Kumar A, Roberts D, Wood KE, et al. Duration of hypotension before initiation of effective antimicrobial therapy is the critical determinant of survival in human septic shock. Crit Care Med 2006;34:1589-96. [Crossref] [PubMed]
- Levy MM, Evans LE, Rhodea A. The Surviving Sepsis Campaign Bundle: 2018 Update. Crit Care Med 2018;46:997-1000. [Crossref] [PubMed]
- IDSA Sepsis Task Force. Infectious Diseases Society of America (IDSA) position statement: Why IDSA Did Not Endorse the Surviving Sepsis Campaign Guidelines. Clin Infect Dis 2018;66:1631-35. [Crossref] [PubMed]
- Kollef MH, Bassetti M, Francois B, et al. The Intensive Care Med research agenda on multidrug-resistant bacteria, antibiotics, and stewardship. Intensive Care Med 2017;43:1187-97. [Crossref] [PubMed]
- Trouillet JL, Vuagnat A, Combes A, et al. Pseudomonas aeruginosa ventilator-associated pneumonia: comparison of episodes due to piperacillin-resistant versus piperacillin-susceptible organisms. Clin Infect Dis 2002;34:1047-54. [Crossref] [PubMed]
- Luyt C-E, Aubry A, Lu Q, et al. Imipenem, meropenem, or doripenem to treat patients with Pseudomonas aeruginosa ventilator-associated pneumonia. Antimicrob Agents Chemother 2014;58:1372-80. [Crossref] [PubMed]
- MacVane SH. Antimicrobial Resistance in the Intensive Care Unit: A Focus on Gram-Negative Bacterial Infections. J Intensive Care Med 2017;32:25-37. [Crossref] [PubMed]
- Munoz-Price LS, Poirel L, Bonomo RA, et al. Clinical epidemiology of the global expansion of Klebsiella pneumoniae carbapenemases. Lancet Infect Dis 2013;13:785-96. [Crossref] [PubMed]
- Maltezou HC, Kontopidou F, Dedoukou X, et al. Action Plan to combat infections due to carbapenem-resistant, Gram-negative pathogens in acute-care hospitals in Greece. J Glob Antimicrob Resist 2014;2:11-6. [Crossref] [PubMed]
- Zusman O, Altunin S, Koppel F, et al. Polymyxin monotherapy or in combination against carbapenem-resistant bacteria: systematic review and meta-analysis. J Antimicrob Chemother 2017;72:29-39. [Crossref] [PubMed]
- Gutiérrez-Gutiérrez B, Salamanca E, de Cueto M, et al. Effect of appropriate combination therapy on mortality of patients with bloodstream infections due to carbapenemase-producing Enterobacteriaceae (INCREMENT): a retrospective cohort study. Lancet Infect Dis 2017;17:726-34. [Crossref] [PubMed]
- Machuca I, Gutiérrez-Gutiérrez B, Gracia-Ahufinger I, et al. Mortality Associated with Bacteremia Due to Colistin-Resistant Klebsiella pneumoniae with High-Level Meropenem Resistance: Importance of Combination Therapy without Colistin and Carbapenems. Antimicrob Agents Chemother 2017;61. [Crossref] [PubMed]
- Lepak AJ, Zhao M, VanScoy B, et al. In Vivo Pharmacokinetics and Pharmacodynamics of ZTI-01 (Fosfomycin for Injection) in the Neutropenic Murine Thigh Infection Model against Escherichia coli, Klebsiella pneumoniae, and Pseudomonas aeruginosa. Antimicrob Agents Chemother 2017;61. [Crossref] [PubMed]
- Shields RK, Potoski BA, Haidar G, et al. Clinical Outcomes, Drug Toxicity, and Emergence of Ceftazidime-Avibactam Resistance Among Patients Treated for Carbapenem-Resistant Enterobacteriaceae Infections. Clin Infect Dis 2016;63:1615-8. [Crossref] [PubMed]
- Bassetti M, Righi E. New antibiotics and antimicrobial combination therapy for the treatment of gram-negative bacterial infections. Curr Opin Crit Care 2015;21:402-11. [Crossref] [PubMed]
- Garnacho-Montero J, Dimopoulos G, Poulakou G, et al. Task force on management and prevention of Acinetobacter baumannii infections in the ICU. Intensive Care Med 2015;41:2057-75. [Crossref] [PubMed]
- Dijkshoorn L, Nemec A, Seifert H. An increasing threat in hospitals: multidrug-resistant Acinetobacter baumannii. Nat Rev Microbiol 2007;5:939-51. [Crossref] [PubMed]
- Garnacho-Montero J, Amaya-Villar R. Multiresistant Acinetobacter baumannii infections: epidemiology and management. Curr Opin Infect Dis 2010;23:332-9. [Crossref] [PubMed]
- Koulenti D, Lisboa T, Brun-Buisson C, et al. Spectrum of practice in the diagnosis of nosocomial pneumonia in patients requiring mechanical ventilation in European intensive care units. Crit Care Med 2009;37:2360-8. [Crossref] [PubMed]
- Bassetti M, Righi E, Esposito S, et al. Drug treatment for multidrug-resistant Acinetobacter baumannii infections. Future Microbiol 2008;3:649-60. [Crossref] [PubMed]
- Jaruratanasirikul S, Wongpoowarak W, Aeinlang N, et al. Pharmacodynamics modeling to optimize dosage regimens of sulbactam. Antimicrob Agents Chemother 2013;57:3441-4. [Crossref] [PubMed]
- Levin AS, Barone AA, Penço J, et al. Intravenous colistin as therapy for nosocomial infections caused by multidrug-resistant Pseudomonas aeruginosa and Acinetobacter baumannii. Clin Infect Dis 1999;28:1008-11. [Crossref] [PubMed]
- Kallel H, Hergafi L, Bahloul M, et al. Safety and efficacy of colistin compared with imipenem in the treatment of ventilator-associated pneumonia: a matched case-control study. Intensive Care Med 2007;33:1162-7. [Crossref] [PubMed]
- Sader HS, Farrell DJ, Flamm RK, et al. Antimicrobial susceptibility of Gram-negative organisms isolated from patients hospitalised with pneumonia in US and European hospitals: results from the SENTRY Antimicrobial Surveillance Program, 2009-2012. Int J Antimicrob Agents 2014;43:328-34. [Crossref] [PubMed]
- Maraolo AE, Cascella M, Corcione S, et al. Management of multidrug-resistant Pseudomonas aeruginosa in the intensive care unit: state of the art. Expert Rev Anti Infect Ther 2017;15:861-71. [Crossref] [PubMed]
- Traugott KA, Echevarria K, Maxwell P, et al. Monotherapy or combination therapy? The Pseudomonas aeruginosa conundrum. Pharmacotherapy 2011;31:598-608. [Crossref] [PubMed]
- Tamma PD, Cosgrove SE, Maragakis LL. Combination therapy for treatment of infections with gram-negative bacteria. Clin Microbiol Rev 2012;25:450-70. [Crossref] [PubMed]
- Bassetti M, Carnelutti A, Peghin M. Patient specific risk stratification for antimicrobial resistance and possible treatment strategies in gram-negative bacterial infections. Expert Rev Anti Infect Ther 2017;15:55-65. [Crossref] [PubMed]
- Boomer JS, Green JM, Hotchkiss RS. The changing immune system in sepsis: is individualized immuno-modulatory therapy the answer? Virulence 2014;5:45-56. [Crossref] [PubMed]
- Harrison C. Sepsis: calming the cytokine storm. Nat Rev Drug Discov 2010;9:360-1. [Crossref] [PubMed]
- Venet F, Monneret G. Advances in the understanding and treatment of sepsis-induced immunosuppression. Nat Rev Nephrol 2018;14:121-37. [Crossref] [PubMed]
- Busani S, Serafini G, Mantovani E, et al. Mortality in Patients With Septic Shock by Multidrug Resistant Bacteria. J Intens Care Med 2017. [Epub ahead of print].
- Becattini S, Taur Y, Pamer EG. Antibiotic-Induced Changes in the Intestinal Microbiota and Disease. Trends Mol Med 2016;22:458-78. [Crossref] [PubMed]
- Girardis M, Cossarizza A. Early alterations of B cells in patients with septic shock: another piece in the complex puzzle of the immune response in sepsis. Crit Care 2013;17:162. [Crossref] [PubMed]
- Nasi M, De Biasi S, Gibellini L, et al. Ageing and inflammation in patients with HIV infection. Clin Exp Immunol 2017;187:44-52. [Crossref] [PubMed]
- Pinti M, Appay V, Campisi J, et al. Aging of the immune system: Focus on inflammation and vaccination. Eur J Immunol 2016;46:2286-301. [Crossref] [PubMed]
- Busani S, Roat E, Serafini G, et al. The Role of Adjunctive Therapies in Septic Shock by Gram Negative MDR/XDR Infections. Can J Infect Dis Med Microbiol 2017;2017:2808203. [Crossref] [PubMed]
- Patil NK, Bohannon JK, Sherwood ER. Immunotherapy: A promising approach to reverse sepsis-induced immunosuppression. Pharmacological Research 2016;111:688-702. [Crossref] [PubMed]
- Francois B, Jeannet R, Daix T, et al. Interleukin-7 restores lymphocytes in septic shock: the IRIS-7 randomized clinical trial. JCI Insight 2018. [Epub ahead of print]. [Crossref] [PubMed]
- Lipson EJ, Forde PM, Hammers HJ, et al. Antagonists of PD-1 and PD-L1 in Cancer Treatment. Semin Oncol 2015;42:587-600. [Crossref] [PubMed]
- Hutchins NA, Unsinger J, Hotchkiss RS, et al. The new normal: immunomodulatory agents against sepsis immune suppression. Trends Mol Med 2014;20:224-33. [Crossref] [PubMed]
- Hotchkiss RS, Moldawer LL, Opal SM, et al. Sepsis and septic shock. Nat Rev Dis Primers 2016;2:16045. [Crossref] [PubMed]
- Clark E, Molnar AO, Joannes-Boyau O, et al. High-volume hemofiltration for septic acute kidney injury: a systematic review and meta-analysis. Crit Care 2014;18:R7. [Crossref] [PubMed]
- Borthwick EMJ, Hill CJ, Rabindranath KS, et al. High-volume haemofiltration for sepsis. Cochrane Database Syst Rev 2013.CD008075. [PubMed]
- Quenot JP, Binquet C, Vinsonneau C, et al. Very high volume hemofiltration with the Cascade system in septic shock patients. Intensive Care Med 2015;41:2111-20. [Crossref] [PubMed]
- Dellinger RP, Bagshaw SM, Antonelli M, et al. Effect of Targeted Polymyxin B Hemoperfusion on 28-Day Mortality in Patients With Septic Shock and Elevated Endotoxin Level: The EUPHRATES Randomized Clinical Trial. JAMA 2018;320:1455-63. [Crossref] [PubMed]
- Cruz DN, Antonelli M, Fumagalli R, et al. Early use of polymyxin B hemoperfusion in abdominal septic shock: the EUPHAS randomized controlled trial. JAMA 2009;301:2445-52. [Crossref] [PubMed]
- Payen DM, Guilhot J, Launey Y, et al. Early use of polymyxin B hemoperfusion in patients with septic shock due to peritonitis: a multicenter randomized control trial. Intensive Care Med 2015;41:975-84. [Crossref] [PubMed]
- Mao HJ, Yu S, Yu XB, et al. Effects of coupled plasma filtration adsorption on immune function of patients with multiple organ dysfunction syndrome. Int J Artif Organs 2009;32:31-8. [Crossref] [PubMed]
- Meisel C, Schefold JC, Pschowski R, et al. Granulocyte-macrophage colony-stimulating factor to reverse sepsis-associated immunosuppression: a double-blind, randomized, placebo-controlled multicenter trial. Am J Respir Crit Care Med 2009;180:640-8. [Crossref] [PubMed]
- Bo L, Wang F, Zhu J, et al. Granulocyte-colony stimulating factor (G-CSF) and granulocyte-macrophage colony stimulating factor (GM-CSF) for sepsis: a meta-analysis. Crit Care 2011;15:R58. [Crossref] [PubMed]
- Döcke WD, Randow F, Syrbe U, et al. Monocyte deactivation in septic patients: restoration by IFN-gamma treatment. Nat Med 1997;3:678-81. [Crossref] [PubMed]
- Hotchkiss RS, Monneret G, Payen D. Sepsis-induced immunosuppression: from cellular dysfunctions to immunotherapy. Nat Rev Immunol 2013;13:862-74. [Crossref] [PubMed]
- Wilson JK, Zhao Y, Singer M, et al. Lymphocyte subset expression and serum concentrations of PD-1/PD-L1 in sepsis - pilot study. Crit Care 2018;22:95. [Crossref] [PubMed]
- Brahmamdam P, Inoue S, Unsinger J, et al. Delayed administration of anti-PD-1 antibody reverses immune dysfunction and improves survival during sepsis. J Leukoc Biol 2010;88:233-40. [Crossref] [PubMed]
- Zhang Y, Zhou Y, Lou J, et al. PD-L1 blockade improves survival in experimental sepsis by inhibiting lymphocyte apoptosis and reversing monocyte dysfunction. Crit Care 2010;14:R220. [Crossref] [PubMed]
- Chang K, Svabek C, Vazquez-Guillamet C, et al. Targeting the programmed cell death 1: programmed cell death ligand 1 pathway reverses T cell exhaustion in patients with sepsis. Crit Care 2014;18:R3. [Crossref] [PubMed]
- Sierro S, Romero P, Speiser DE. The CD4-like molecule LAG-3, biology and therapeutic applications. Expert Opinion on Therapeutic Targets 2011;15:91-101. [Crossref] [PubMed]
- Busani S, Damiani E, Cavazzuti I, et al. Intravenous immunoglobulin in septic shock: review of the mechanisms of action and meta-analysis of the clinical effectiveness. Minerva Anestesiol 2016;82:559-72. [PubMed]
- Bermejo-Martín JF, Rodriguez-Fernandez A, Herrán-Monge R, et al. Immunoglobulins IgG1, IgM and IgA: a synergistic team influencing survival in sepsis. J Intern Med 2014;276:404-12. [Crossref] [PubMed]
- Giamarellos-Bourboulis EJ, Tziolos N, Routsi C, et al. Improving outcomes of severe infections by multidrug-resistant pathogens with polyclonal IgM-enriched immunoglobulins. Clin Microbiol Infect 2016;22:499-506. [Crossref] [PubMed]
- Ainsworth S, Ketter PM, Yu JJ, et al. Vaccination with a live attenuated Acinetobacter baumannii deficient in thioredoxin provides protection against systemic Acinetobacter infection. Vaccine 2017;35:3387-94. [Crossref] [PubMed]
- Zhang X, Yang T, Cao J, et al. Mucosal immunization with purified OmpA elicited protective immunity against infections caused by multidrug-resistant Acinetobacter baumannii. Microb Pathog 2016;96:20-5. [Crossref] [PubMed]
- Sawa T, Ito E, Nguyen VH, et al. Anti-PcrV antibody strategies against virulent Pseudomonas aeruginosa. Hum Vaccin Immunother 2014;10:2843-52. [Crossref] [PubMed]
- Okada M, Kita Y, Hashimoto S, et al. Preclinical study and clinical trial of a novel therapeutic vaccine against multi-drug resistant tuberculosis. Hum Vaccin Immunother 2017;13:298-305. [Crossref] [PubMed]
Cite this article as: Tosi M, Roat E, De Biasi S, Munari E, Venturelli S, Coloretti I, Biagioni E, Cossarizza A, Girardis M. Multidrug resistant bacteria in critically ill patients: a step further antibiotic therapy. J Emerg Crit Care Med 2018;2:103.