Advances in lung ultrasound in critically ill patients
Introduction
In the last 15 years, transthoracic lung ultrasound (LUS) has proven to be one of the most revolutionary diagnostic tools in the intensive care environment (1) where acute respiratory failure is the most frequent cause of hospitalisation with a mortality rate of 33–37% among those requiring invasive mechanical ventilation (2). Its great success is due to it being minimally invasive, easily repeated and simple to carry out. Furthermore, transthoracic LUS only requires a rapid learning curve; 25 supervised tests are sufficient to achieve a basic knowledge (3). Moreover, the impact of transthoracic LUS can lead to important clinical decisions being made in about 50% of intervention cases: bronchoscopy, chest tube placement, positive end-expiratory pressure (PEEP) titration, recruitment manoeuvre, fluid management, diuretics, antibiotic initiation/change, physiotherapy (4). Additionally, the early use of scoring such as the “LUS score” is helpful in predicting the outcome of patients admitted to the intensive care unit (ICU) (5). With its image artefacts (A-lines, B-lines) or direct images (lung consolidations, pleural effusions), LUS provides significant information that can be integrated with the clinical signs and symptoms of the critically ill patient. In the following paper, we will focus on four of the major aspects that have revolutionised our clinical practice in recent years, each one making a huge impact on patient care (Figure 1). This review is aimed at those doctors who are already using transthoracic LUS in daily clinical practice, assisting them in focusing on certain precise aspects, specifically diagnosis, prognosis and future haemodynamic applications.
LUS score
LUS examination is primarily based on the evaluation of artefacts, since ultrasound is not transmitted through aerated interfaces. LUS may appear simple but needs to be performed with rigorous methods to produce as much reliable information as possible (6,7). Different features of LUS patterns have been described and most relate to the aeration level of the parenchyma (8). Typical findings of LUS examinations are the so-called “A-lines”, a reverberation artefact of a pleural line spreading through aerated lung parenchyma, and the visualisation of pleural sliding, the relative mutual movement of visceral and parietal pleura during the inspiration (9). Pathological findings relate to diminished aerated parenchyma. “B-lines” represent the decrease in air content of the lung parenchyma and—in the appropriate clinical picture—reflect the presence of extravascular lung water (EVLW) which alters the gas-tissue interface and produces comet-tail artefacts moving from the pleural line to deep parenchyma (7,10). The presence of B-lines implies a moderate reduction of lung aeration, which is typical of interstitial syndrome (11). Lung consolidation consists of a major loss of aerated parenchyma (i.e., lobar pneumonia, pulmonary contusion or atelectasis), which appears as a tissue-like texture. The shred sign, suggestive of an indented pleural line around the lung consolidation, and the dynamic air bronchogram, caused by air penetration in the bronchial tree but not in the alveolar space, better defines the aerated parenchyma mass loss (12,13).
Lung aeration is therefore an important aspect to consider in optimising the ventilatory parameters and in monitoring a successful weaning process in order not to lose aerated parenchymal mass. In this context, LUS examination is a structured, standardized and comprehensive evaluation of pulmonary parenchyma that aims to help clinicians monitor lung aeration (14). Indeed, LUS patterns provide a satisfying picture of the aerated parenchyma and any variation can be effectively translated into a score. Therefore, LUS evaluation starts with the identification of three different areas of the thorax, anterior, lateral and posterior regions, divided by anterior and posterior axillary lines as anatomical landmarks. Each area is further divided into superior and inferior, thus defining six specific sections per hemithorax (Figure 2). Four different aeration patterns are assigned to each region: (I) presence of A-line artefacts (A), typical of normal aeration; (II) prevalence of B-line patterns (B1), suggesting a limited decrease in lung aeration (i.e., interstitial syndrome); (III) confluent B-lines (B2), suggesting a more severe loss of aerated lung due to liquid imbibition of the alveolar space (i.e., pulmonary oedema); (IV) complete lung consolidation (C), with hepatisation of lung parenchyma and dynamic air bronchogram, suggesting major loss of aerated lung mass. The LUS is then calculated on the basis of the worst ultrasound pattern detected in each region, whereby A =0 points, B1 =1 point, B2 =2 points, C =3 points. The total LUS score comes from the sum of each region’s points, ranging from 0 to 36 (15,16).
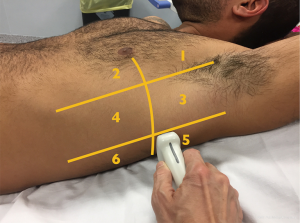
The LUS score helps clinicians to obtain a detailed screenshot of aerated parenchymal mass and it also provides detailed information of clinical relevance (9). Where the role of LUS in diagnosing pneumothorax is a recognised practice, more recent and fascinating roles are its ability to optimise ventilatory settings in adult respiratory distress syndrome (ARDS) patients, estimate the recruitable, poorly aerated pulmonary mass, monitor lung aeration during the weaning process, and give a reliable prognostic factor.
In ARDS patients the aeration loss typically strikes focal regions of parenchyma, focusing mainly on the lower lobes, while upper regions may remain normally aerated (17). LUS patterns in ARDS consist of both B-lines and consolidation (18). Since lung recruitment involves particularly poorly aerated areas rather than consolidated regions (5), an LUS score allows clinicians to quantify the relative share between these two patterns, helping to set appropriate mechanical ventilation parameters, balance alveolar recruitment and reduce the risk of lung overinflation.
On the other hand, other clinical features, such as pulmonary oedema, are characterized by multiple and confluent B-lines throughout all examined areas, due to an increase of intravascular hydrostatic pressure (18,19). This condition benefits from positive-pressure ventilation, which rebalances hydrostatic pressure between the intravascular and interstitial spaces. In this context, the LUS score best defines recruitment areas (20) and may guide incremental PEEP trials in order to restore a normal pulmonary pattern (15).
Moreover, the LUS score acts as a useful tool to monitor lung aeration during the weaning process. Failed extubation complicates 3–30% of successful spontaneous breathing trials (21), causes a longer stay in ICU (22), increases the risk of ventilator-associated pneumonia (23), and has higher morbidity and mortality rates (24). The LUS score shows how switching from positive to negative pressure produces better aeration of the lower posterior regions, while in the upper anterior and lateral areas it may decrease. The reduced aeration rate during a spontaneous breathing trial may reveal its outcome (16).
Finally, the LUS score is acquiring greater prominence for its prognostic implications. LUS scores are claimed to correlate with the severity of a disease and mortality rates of ARDS patients. In particular, the number of B-lines at ultrasound evaluation seems to directly correlate to mortality (25), while the LUS score relates to EVLW, measured by the transpulmonary thermodilution technique (5). This means LUS is a non-invasive, real-time, radiation-free, bedside method for the early diagnosis and correct prognosis of ARDS patients, suggesting its use as an alternative to radiology in the diagnostic process (Table 1).
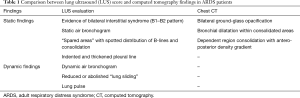
Full table
Fluid management with LUS
The administration of fluids in ICU is the cornerstone in the treatment of critical ill patients (26). Over time this clinical practice has become common sense, associated with a positive feeling of doing something good for the patient. However, recent scientific evidence points out that excessive fluid balance is associated with a worse outcome for the patient (27-29), and, for example, in the septic patient, a positive fluid balance and a high central venous pressure (CVP) have been associated with an increase in mortality (30). Meanwhile, other studies have shown that administering fewer fluids is better (31-34). The FENICE trial brought attention to a “safety problem” due to the fact that only 59% of patients showed fluid responsiveness while the other 41% were exposed to the problem of “overhydration” (35). The last fifteen years have also been characterised by a decline in the invasive haemodynamic monitoring of cardiac output (CO) (36) and a reduced use of semi-invasive or non-invasive techniques due to their intrinsic limitations (37-39). Conversely, there has been an explosion in the use of point-of-care ultrasonography in terms of critical care echocardiography (CCE) and transthoracic LUS (40). So far, things have shifted from numbers, i.e., CO, CVP, wedge pressure, to “visual thinking”, i.e., ejection fraction “eyes ball” assessment for the heart and “A-line profile” and “B-line profile” and so forth for the lungs (40,41). In other words, we have learned that “diffuse” bilateral B-lines instead of “focal” multiple B-lines (i.e., pneumonia) in acute clinical conditions are very often associated with increased EVLW due to a possible systolic or diastolic cardiogenic shock with elevated pulmonary pressures (Figure 3), or to damaged pulmonary vessels (glycocalyx), secondary to the ARDS (42-44). In both cases the lung is “wet” (B-profile) and not “dry” (A profile). It has also been proven that after a fluid bolus, B-lines increase by 8%, with a heterogeneous distribution in the lung and aeration loss, even if haemodynamic variables return to the previous values as a result of fluid redistribution from the vascular compartment (20,45). In other words, the haemodynamic benefits of fluid loading may be associated with worsened lung aeration, and B-lines seem to be directly related to EVLW and higher mortality (20,45,46). In published articles, the recommendations for fluid loading are clear: to be liberal at the initial phase of septic shock, possibly in the first 2 hours (47), because fluid restriction can worsen the function of the organs (33). LUS could today play an interesting role in fluid management due to the fact that static variables, i.e., CVP, pulmonary arterial occlusion pressure (PAOP), intrathoracic blood volume (ITBV), and so forth, and dynamic indices of preload, i.e., systolic arterial pressure (SAP), pulse pressure variation (PPV), and inferior vena cava (IVC) variation, have shown many limitations (41-42). Combining LUS with CCE can provide highly relevant information (Figure 4) in terms of fluid “tolerance” or fluid “intolerance”. In the first case, a diffuse bilateral “A-profile” (dry lung) means that the patient shows fluid tolerance (43). However, this is not a guarantee that the patient needs fluid, and this is the same limitation shown by dynamic preload indices (44). In the second case, when a diffuse “B-profile” from the base to the apex is shown (wet lung), it is important to stop fluids and think about norepinephrine in those patients who remain hypotensive [mean arterial pressure (MAP) <65 mmHg] after having a proper ultrasound heart examination (20,45). Usually, CO can be estimated with a non-invasive transthoracic echocardiography, as the product of heart rate (HR) and stroke volume (SV), where SV is equal to the product of the velocity time integral (VTI) and the aortic cross section area (CSA). CSA is equal to π (dLVOTcm/2)2. To obtain CSA = π (dLVOTcm/2)2, the diameter of the left ventricular outflow tract (LVOT) from the parasternal window needs to be acquired, where even the smallest error becomes squared and can generate discrepancies in CO evaluation. To overcome this limitation, the VTI is considered a repeatable measurement that can be obtained in the apical five chamber view using pulsed waves. Normal values for VTI are between 18 and 22 cm. The combination of transthoracic LUS and CCE in this context may allow clinicians to obtain additional information regarding the effect of fluid loading on patients haemodynamic (Figure 4).
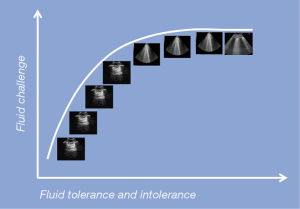
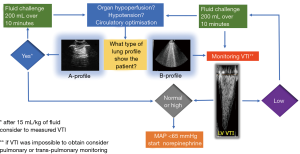
The Surviving Sepsis Campaign continues to recommend an empiric fluid bolus of 30 mL/kg for all patients presenting with hypotension or an elevated lactate level, independent of the lung “intolerance” (47). However, there is no clear evidence that substantial fluid resuscitation reliably improves end-organ perfusion in septic patients. A recent study discovered that in adult patients presenting with septic shock, early fluid boluses caused harm (27-30,46). Among intensive care physicians transthoracic LUS is becoming a useful and non-invasive tool to observe the lung’s fluid refractory or fluid “intolerance”. Some data has also suggested that a restricted fluid approach would be prudent in patients with sepsis to reduce morbidity and improve the outcome (46).
Pleural effusion
Pleural effusion is a common pathological condition among critical care patients. At the time of admission into ICU, 41% of patients are diagnosed with pleural effusion, while 21% will develop it during hospitalisation (48). Because pleural effusion can worsen gas exchange, respiratory mechanics and hemodynamic stability (48,49), an early diagnosis of the presence and quality of the pleural effusion is necessary to adopt adequate therapeutic, invasive or non-invasive strategies. The gold standard for the diagnosis of pleural effusion remains the computed tomography (CT), which requires the patient to be moved out of intensive care, not always an easy task (50). Transthoracic LUS allows a bedside approach for pleural effusion diagnosis with a sensitivity of 92%, a specificity of 93% and a diagnostic accuracy of 93% (51), without ionising radiation. Transthoracic LUS accuracy has proven to be higher than anteroposterior or lateral chest X-rays (52,53), capable of identifying the presence of effusions smaller than 15 mm (53). Therefore, the International Consensus Conference on Lung Ultrasound states, “For the detection of effusion, transthoracic LUS is more accurate than supine radiography and is as accurate as CT (strong: level A)” (7). Transthoracic LUS can also help clinicians to distinguish between the different types of effusion (transudate, exudate, empyema and haemothorax) by analysing the pleural effusion’s internal echogenicity and changes in lung parenchyma and pleural thickness (>3 mm) (54). Transudates and exudates can both appear as homogeneous anechoic pleural effusions: clinical contexts and visualisation of internal echoes (mobile particles and/or septation) suggest exudates; changes in the appearance of the lung parenchyma (e.g., consolidation) and an increase in pleural thickness can also lead clinicians to identify an exudate pleural effusion (54,55). Empyema and haemothorax can both appear as homogeneous echogenic effusions (54). Transthoracic LUS also enables fluid quantification of pleural effusions and decisions can be made about a therapeutic approach; alterations in gas exchange and fluid volume are the most important considerations when deciding whether or not to drain the pleural effusion. Benefits of pleural drains should be considered against the risk of complications (pneumothorax, haemothorax, visceral injury). Despite reliable estimations of the effusion, its volume remains challenging for various reasons, including a non-established best method, size and position of the thoracic cavity, position of the patient, diaphragm dysfunction, very large pleural effusions, concomitant collapsed lung and lung consolidation (56-60) (Table 2). Of course, a greater thickness of effusion ensures a greater safety margin during both invasive diagnostic (pleural aspiration) and therapeutic (pleural drain insertion) procedures (63). Regarding this safety margin, The British Thoracic Society Pleural Disease Guideline 2010 states, “When using ultrasound to select a site for aspiration of a pleural effusion, the site chosen should have (I) sufficient depth of pleural fluid (at least 10 mm), (II) no intervening lung at maximal inspiration and (III) minimal risk of puncture of other structures such as the heart, liver and spleen” (63). Small effusions (<10 mm thickness) can usually be resolved with non-invasive therapeutic strategies alone (diuretics, antibiotics) (63). Transthoracic LUS will identify the best puncture site for invasive manoeuvres (thoracentesis and pleural drain insertion) to increase periprocedural efficacy and safety and decrease life-threatening complications (64,65). The best puncture site is where the physician can safely identify each anatomical structure (diaphragm, organs, intercostal artery), or the maximum distance between the visceral and parietal pleura (safety margin) (63,66). Despite non-ultrasound guides, pleural drain insertions must be performed into the safety triangle, the physician being guided by the ultrasound (66). It was once thought that ultrasound could not be employed for intercostal artery visualisation (63). However, several trials have provided evidence about how thoracic Doppler ultrasound can be used to identify intercostal vessels (67-69) for the prevention of bleeding, even in patients with altered coagulation parameters (70). The superiority of ultrasound to detect the best puncture site and reduce potential complications has been confirmed by several studies (64,65), therefore, The British Thoracic Society Pleural Disease Guideline 2010 states, “Site selection for all pleural aspiration should be ultrasound guided” (63). Ultrasound guidance in pleural procedures is performed using both “site marking” and “direct needle guidance”. In the former the physician defines the optimal site with a mark on the skin before puncture, which avoids repositioning the patient as this will cause fluid to move. Direct needle guidance is more technically challenging, however, it permits a needle’s tip real-time visualisation and also guidewire and drainage final position during small-bore pleural drain insertion (66). Transthoracic LUS allows the detection of possible complications after invasive procedures (e.g., pneumothorax, bleeding, see pneumothorax section). Transthoracic LUS also permits the identification of residual pleural effusions despite a poorly supplied pleural drain. The pleural drain should be removed when it drains less than 200 mL per day, the pneumothorax is resolved, or when the drain is no longer functioning (63). Therefore, due to its high accuracy in diagnosing pneumothorax and pleural effusions, transthoracic LUS also provides useful indications about when to remove drains. Moreover, transthoracic LUS identifies co-existing lung diseases, with a higher specificity and sensitivity than chest radiography (pneumothorax, interstitial syndrome, consolidation and atelectasis) (51). As critically ill intensive care patients may have limited mobility, and more recumbent positions can lead to haemodynamic side effects, we suggest a supine position with a mild torso elevation of 15°. In this position all free fluids are collected by gravity at the PLAPS point (posterolateral alveolar and/or pleural syndrome) and so a scan of this PLAPS point can detect and quantify the fluid in the pleural effusion, even small ones (71), using Balik’s formula (58). More recumbent patient positions can also lead to overestimating the volume of a pleural effusion (58,60). Due to its bedside approach, thoracic LUS, in the case of pleural effusions, could be essential, from the initial diagnosis, throughout the clinical course, to the final therapeutic treatment (6). We suggest that a thoracic LUS evaluation is performed upon admission of the patient to ICU and re-evaluated daily, or after clinical changes/invasive procedures are performed.
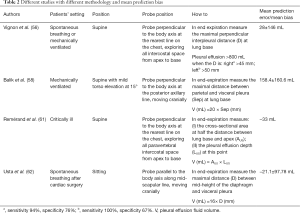
Full table
Pneumothorax
The accumulation of air in the pleural cavity causes a condition known as pneumothorax. The amount and rate at which air enters the pleural space (usually virtual) can cause haemodynamic effects as the compression of the large venous vessels inside the mediastinum (as well as the stretching of the parenchymatous structures) determines a life-threatening condition called tension pneumothorax. In critical care medicine, in ICU as well as in the emergency department or a pre-hospital environment, excluding pneumothorax as a cause of a respiratory distress and/or a haemodynamic shock is essential to enable life-saving procedures (emergency chest decompression and thoracic drainage) (71-74). The gold standard test for the diagnosis of pneumothorax is CT. However, because of the need for a quicker diagnosis, often at the bedside (also because of the non-optimal diagnostic accuracy of the chest radiograph), the use of transthoracic LUS as a diagnostic test for pneumothorax has progressively spread. The sensitivity and specificity of transthoracic LUS in the diagnosis of pneumothorax have been analysed extensively in published articles: sensitivity is around 80–90% and specificity is around 98% (75-79). The sensitivity of the chest radiograph, on the contrary, is about 40–50%. In fact, there is a wide limitation in the diagnostic accuracy of this method linked to the position of the patient and the masking due to bone structures. Since air tends to accumulate in the highest points of the thorax (i.e., in the anti-gravity positions), in the supine patient (as the critically ill patient is most frequently placed), the pneumothorax, even in small cases, is more easily identifiable in the parasternal areas of the thorax, and anteriorly and superiorly in the chest. Visualising at least two ribs longitudinally and the pleural line between the two, allows clinicians to quickly obtain a reference point of the echogenicity and the movement of the pleural line. The probe is then rotated 90° to gain a better visualisation of the pleural line, before eventually zooming in on it. Once the pneumothorax has been identified, it is advised to gradually move the probe towards the axillary line to establish, at least roughly, the dimensions of the pneumothorax (see further on the meaning of the lung point) (71). There is no data in published articles to suggest that the linear probe is better than the convex probe (80). Several sonographic signs have been tested in recent years, including the absence of lung sliding, B-lines or comet-tail artefacts, A-lines, and the lung point sign (81). Lung sliding is an ultrasound sign quite easily identifiable in the healthy chest. However, when air separates the two pleural sheets, the visceral pleura becomes invisible to the ultrasound beam. Sonographically we can detect the presence of the pleural line, which in this case is immobile, signifying that there is no lung sliding. This artefact is detectable in both B-mode and M-mode. The absence of lung sliding does not have a high specificity since other conditions, such as atelectasis, pulmonary contusion or a main stem intubation, can determine the same sonographic sign (81). The presence of even one B-line is virtually able to exclude the pneumothorax. In fact, the genesis of this artefact is linked to the phenomena of multiple refraction of the ultrasound beam inside the parenchyma due to the strong difference in impedance between two surfaces. The B-lines originate from the pleural line and have a vertical course within the ultrasound field. They move synchronised with respiratory movements. Because of their nature, the B-lines are not visible if a high-impedance surface, such as air, is interposed between the two pleural sheets. Therefore, the visualisation of one or more B-lines indicates the adherence of the two pleurae and totally excludes the presence of pneumothorax. However, the absence of B-lines does not prove the presence of pneumothorax, this being the normal condition of the healthy lung, besides several pathological conditions (81). In the lung zones next to the heart it is possible to identify an alternative movement to pleural sliding, which consists of a wobbling motion of the pleural line synchronised with the cardiac rhythm. This sign is called the lung pulse. The lung pulse is virtually absent in pneumothorax, unlike other pathological conditions (such as atelectasis), because the amount of air between the pleural sheets prevents the transmission of mechanical oscillation. This sign rules out the presence of pneumothorax, fulfilling the same role of the B-lines. Compared to the signs discussed so far, the lung point, the point where the two pleural sheets come back to each other and therefore lung sliding reappears, if present, confirms the diagnosis of pneumothorax (81). Intuitively, the greater the distance from the hilum (and therefore from the anterior axillary line) to the lung point, the more extensive the pneumothorax. Finding a lung point beyond the medium axillary line predicts (with a sensitivity and specificity of about 80%) a collapse of more than 15% of the lung (82). However, the overall sensitivity in diagnosing pneumothorax by the lung point is very low. In fact, in the case of a complete collapse of the lung, the lung point is absent, since there are no points where the pleura come back to each other. Moreover, in the case of pleural blebs or pleural adhesions that determine the formation of a saccade, the presence of a lung point may not indicate an extension of the pneumothorax (83-85). Sometimes this condition is associated with the so-called “double lung point” (86). Summarising the role of sonographic signs in the diagnosis of pneumothorax, we can state the following: the first sign to be sought is lung sliding (87). If lung sliding is absent, it is useful to look for the presence of at least one B-line. If there is no B-line, pneumothorax cannot be ruled out. If, at this step, we identify the lung point we can diagnose pneumothorax (71) (Figure 5). Ultrasound is, at present, an extremely useful tool for ruling in or out the diagnosis of pneumothorax in critically ill patients and therefore it is able to rapidly contribute to potentially life-saving therapeutic decisions.
Limitations of LUS
In the intensive care setting, over the years, no monitoring system has been shown to change patients’ outcomes. This is also true of transthoracic LUS. However, with brief training, this tool can be used to detect the severity of lung disease after ICU admission and to monitor the progress of the disease without invasive procedures. Furthermore, the detection of different lung profiles—wet or dry—can facilitate patient diagnosis in the clinical context. If it is true that lung fluid tolerance is a futile finding in the stable patient, because it is no guarantee that the patient needs fluid, lung refractory or fluid intolerance is a very important step in haemodynamic monitoring, albeit requiring competence in CCE. Furthermore, we need to acknowledge the fact that aortic stenosis can preclude this step due to the overestimation of VTI. In this case a haemodynamic invasive monitoring such as the pulmonary or transpulmonary thermodilution technique could be required.
Unfortunately, at present, there is no validated method for establishing the size of a pneumothorax using transthoracic LUS alone. This is very important considering that the current guidelines of the main international scientific societies establish different therapeutic approaches on the basis (besides the traumatic origin of the pneumothorax) of the dimensions of the pneumothorax (the different guidelines agree with each other for no more than 40%) (88) (Table 3). In addition to the limits already described, in the case of emphysematous bubbles that can simulate a pneumothorax (the difference of which, however, may be the presence of a second lung point beyond the first, i.e., double lung point), the biggest limitation in the use of ultrasound in the diagnosis of pneumothorax is the presence of subcutaneous emphysema. In this case, ultrasound can detect vertical linear artefacts, called “E-lines”, that could be confused with B-lines, but instead start from the subcutaneous tissue. To correctly distinguish between these two artefacts, it is important to identify the space between two ribs in order to visualise the pleural line. All in all, and with this limitation in mind, we need to remember that transthoracic LUS is a powerful instrument but that diagnosis and subsequent therapeutic decisions must be made on the basis of the clinical context, the patient’s history and, ultimately, the entire clinical picture.

Full table
Future perspectives for LUS
- We hope that in the future LUS scores would gain greater attention in the ARDS definition and classification, specifically in light of its powerful role to correlate the severity of the disease with the outcome;
- According to several studies (56,58,61,62), the use of transthoracic LUS to estimate pleural fluid requires urgent standardisation, and we hope that given the clinical importance of estimating pleural effusion this point will be discussed in the new version of the International Consensus Conference on Lung Ultrasound Guidelines;
- Although haemodynamic management represents the second most important step after antibiotic prescription in septic patients, transthoracic LUS fluid refractory (intolerance) needs to be recognised as an important step in the management of a patient’s fluid, and that the haemodynamic monitoring of the circulatory system is just a piece of the puzzle. To use a metaphor, it seems that we have to search the “key beneath a lamp” (haemodynamic) because “that is where I can see” (89);
- Transthoracic LUS has tremendous potential in the evaluation and immediate management of critically ill patients at the bedside. Just as, for many years now, stethoscope has become an indispensable tool (i.e., in emergency medicine, cardiology), thoracic LUS could also become an indispensable tool for the intensivist to make fundamental decisions in the management of critically ill patients.
Conclusions
Advances in transthoracic LUS in critical care incorporate physical examinations and visual thinking strategies in a way that offers many opportunities for both the patient and the physician in terms of early goal-directed monitoring and therapy. The application of transthoracic LUS as a score of lung gravity or recovery in ARDS patients, for the assessment and drainage of pleural effusions, for the discovery of a suspected pneumothorax, or for the evaluation of lung fluid intolerance is now well established. As a consequence, transthoracic LUS appears to be a powerful tool in the hands of a trained intensivist, and, at present, to avoid using it seems unjustified.
Acknowledgments
None.
Footnote
Conflicts of Interest: The authors have no conflicts of interest to declare.
Ethical Statement: The authors are accountable for all aspects of the work in ensuring that questions related to the accuracy or integrity of any part of the work are appropriately investigated and resolved.
References
- Liao SF, Chen PJ, Chaou CH, et al. Top-cited publications on point-of-care ultrasound: the evaluation of research trends. Am J Emerg Med 2018;36:1429-38. [Crossref] [PubMed]
- Stefan MS, Shieh MS, Pekow PS, et al. Epidemiology and outcomes of acute respiratory failure in the United States, 2001 to 2009: A national survey. J Hosp Med 2013;8:76-82. [Crossref] [PubMed]
- Rouby JJ, Arbelot C, Gao Y, et al. Training for Lung Ultrasound Score Measurement in Critically Ill Patients. Am J Respir Crit Care Med 2018. [Epub ahead of print]. [Crossref] [PubMed]
- Xirouchaki N, Kondili E, Prinianakis G, et al. Impact of lung ultrasound on clinical decision making in critically ill patients. Intensive Care Med 2014;40:57-65. [Crossref] [PubMed]
- Zhao Z, Jiang L, Xi X, et al. Prognostic value of extravascular lung water assessed with lung ultrasound score by chest sonography in patients with acute respiratory distress syndrome. BMC Pulm Med 2015;15:98. [Crossref] [PubMed]
- Lichtenstein DA, Mezière GA. The BLUE-points: three standardized points used in the BLUE-protocol for ultrasound assessment of the lung in acute respiratory failure. Crit Ultrasound J 2011;3:109-10. [Crossref]
- Volpicelli G, Elbarbary M, Blaivas M, et al. International Liaison Committee on Lung Ultrasound (IL C-LUS) for International Consensus Conference on Lung Ultrasound (ICC-LUS): International evidence-based recommendations for point-of-care lung ultrasound. Intensive Care Med 2012;38:577-91. [Crossref] [PubMed]
- Lichtenstein DA, Mezière GA. Relevance of lung ultrasound in the diagnosis of acute respiratory failure: the BLUE protocol. Chest 2008;134:117-25. [Crossref] [PubMed]
- Bouhemad B, Mongodi S, Via G, et al. Ultrasound for "lung monitoring" of ventilated patients. Anesthesiology 2015;122:437-47. [Crossref] [PubMed]
- Picano E, Frassi F, Agricola E, et al. Ultrasound lung comets: a clinically useful sign of extravascular lung water. J Am Soc Echocardiogr 2006;19:356-63. [Crossref] [PubMed]
- Volpicelli G, Mussa A, Garofalo G, et al. Bedside lung ultrasound in the assessment of alveolar-interstitial syndrome. Am J Emerg Med 2006;24:689-96. [Crossref] [PubMed]
- Lichtenstein DA, Lascols N, Mezière G, et al. Ultrasound diagnosis of alveolar consolidation in the critically ill. Intensive Care Med 2004;30:276-81. [Crossref] [PubMed]
- Lichtenstein D, Mezière G, Seitz J. The dynamic air bronchogram. An ultrasound sign of alveolar consolidation ruling out atelectasis. Chest 2009;135:1421-5. [Crossref] [PubMed]
- Bouhemad B, Liu ZH, Arbelot C, et al. Ultrasound assessment of antibiotic-induced pulmonary reaeration in ventilator-associated pneumonia. Crit Care Med 2010;38:84-92. [Crossref] [PubMed]
- Bouhemad B, Brisson H, Le-Guen M, et al. Bedside ultrasound assessment of positive end-expiratory pressure-induced lung recruitment. Am J Respir Crit Care Med 2011;183:341-7. [Crossref] [PubMed]
- Soummer A, Perbet S, Brisson H, et al. Lung Ultrasound Study Group. Ultrasound assessment of lung aeration loss during a successful weaning trial predicts postextubation distress. Crit Care Med 2012;40:2064-72. [Crossref] [PubMed]
- Copetti R, Soldati G, Copetti P. Chest sonography: a useful tool to differentiate acute cardiogenic pulmonary edema from acute respiratory distress syndrome. Cardiovasc Ultrasound 2008;6:16. [Crossref] [PubMed]
- Bouhemad B, Zhang M, Lu Q, et al. Clinical review: Bedside lung ultrasound in critical care practice. Crit Care 2007;11:205. [Crossref] [PubMed]
- Volpicelli G, Melniker LA, Cardinale L, et al. Lung ultrasound in diagnosing and monitoring pulmonary interstitial fluid. Radiol Med 2013;118:196-205. [Crossref] [PubMed]
- Agricola E, Bove T, Oppizzi M, et al. “Ultrasound comet-tail images”: a marker of pulmonary edema: a comparative study with wedge pressure and extravascular lung water. Chest 2005;127:1690-5. [Crossref] [PubMed]
- Boles JM, Bion J, Connors A, et al. Weaning from mechanical ventilation. Eur Respir J 2007;29:1033-56. [Crossref] [PubMed]
- Kulkarni AP, Agarwal V. Extubation failure in intensive care unit: predictors and management. Indian J Crit Care Med 2008;12:1-9. [Crossref] [PubMed]
- Torres A, Gatell JM, Aznar E, et al. Re-intubation increases the risk of nosocomial pneumonia in patients needing mechanical ventilation. Am J Respir Crit Care Med 1995;152:137-41. [Crossref] [PubMed]
- Peñuelas O, Frutos-Vivar F, Fernández C, et al. Ventila Group. Characteristics and outcomes of ventilated patients according to time to liberation from mechanical ventilation. Am J Respir Crit Care Med 2011;184:430-7. [Crossref] [PubMed]
- Frassi F, Gargani L, Tesorio P, et al. Prognostic value of extravascular lung water assessed with ultrasound lung comets by chest sonography in patients with dyspnea and/or chest pain. J Card Fail 2007;13:830-5. [Crossref] [PubMed]
- Cosnett JE. The origins of intravenous fluid therapy. Lancet 1989;1:768-71. [Crossref] [PubMed]
- Finfer S, Myburgh J, Bellomo R. Intravenous fluid therapy in critically ill adults. Nat Rev Nephrol 2018;14:541-57. [Crossref] [PubMed]
- Durairaj L, Schmidt GA. Fluid therapy in resuscitated sepsis: less is more. Chest 2008;133:252-63. [Crossref] [PubMed]
- Vaara ST, Korhonen AM, Kaukonen KM, et al. FINNAKI Study Group. Fluid overload is associated with an increased risk for 90-day mortality in critically ill patients with renal replacement therapy: data from the prospective FINNAKI study. Critical Care 2012;16:R197. [Crossref] [PubMed]
- Sakr Y, Rubatto Birri PN, Kotfis K, et al. Higher Fluid Balance Increases the Risk of Death From Sepsis: Results From a Large International Audit. Crit Care Med 2017;45:386-94. [Crossref] [PubMed]
- Boyd JH, Forbes J, Nakada TA, et al. Fluid resuscitation in septic shock: a positive fluid balance and elevated central venous pressure are associated with increased mortality. Crit Care Med 2011;39:259-65. [Crossref] [PubMed]
- Maitland K, Kiguli S, Opoka RO, et al. FEAST Trial Group. Mortality after fluid bolus in african children with severe infection. N Engl J Med 2011;364:2483-95. [Crossref] [PubMed]
- Andrews B, Semler MW, Muchemwa L, et al. Effect of an Early Resuscitation Protocol on In-hospital Mortality Among Adults With Sepsis and Hypotension: A Randomized Clinical Trial. JAMA 2017;318:1233-40. [Crossref] [PubMed]
- Brotfain E, Koyfman L, Toledano R, et al. Positive fluid balance as a major predictor of clinical outcome of patients with sepsis/septic shock after ICU discharge. Am J Emerg Med 2016;34:2122-6. [Crossref] [PubMed]
- Cecconi M, Hofer C, Teboul JL, et al. Fluid challenges in intensive care: the FENICE study: A global inception cohort study. Intensive Care Med 2015;41:1529-37. Erratum in: Intensive Care Med 2015;41:1737-8. multiple investigator names added. [Crossref] [PubMed]
- Ikuta K, Wang Y, Robinson A, et al. National trends in use and outcomes of pulmonary artery catheters among medicare beneficiaries 1999-2013. JAMA Cardiol 2017;2:908-13. [Crossref] [PubMed]
- Vetrugno L, Costa MG, Spagnesi L, et al. Uncalibrated arterial pulse cardiac output measurements in patients with moderately abnormal left ventricular function. J Cardiothorac Vasc Anesth 2011;25:53-8. [Crossref] [PubMed]
- Costa MG, Chiarandini P, Scudeller L, et al. Uncalibrated continuous cardiac output measurement in liver transplant patients: LiDCOrapid™ system versus pulmonary artery catheter. J Cardiothorac Vasc Anesth 2014;28:540-6. [Crossref] [PubMed]
- Monnet X, Guérin L, Jozwiak M, et al. Pleth variability index is a weak predictor of fluid responsiveness in patients receiving norepinephrine. Br J Anaesth 2013;110:207-13. [Crossref] [PubMed]
- Vignon P, Peressé X, Vieillard-Baron A, et al. Critical care ultrasonography in acute respiratory failure. Critical Care 2016;20:228. [Crossref] [PubMed]
- Narula J, Chandrashekhar Y, Braunwald E. Time to add a fifth pillar to bedside physical examination inspection, palpation, percussion, auscultation. JAMA Cardiol 2018;3:346-50. [Crossref] [PubMed]
- Laursen CB, Sloth E, Lassen AT, et al. Point-of-care ultrasonography in patients admitted with respiratory symptoms: a single-blind, randomized controlled trial. Lancet Respir Med 2014;2:638-46. [Crossref] [PubMed]
- Lichtenstein D, Mézière G, Biderman P, et al. The comet-tail artifact. An ultrasound sign of alveolar-interstitial syndrome. Am J Respir Crit Care Med 1997;156:1640-6. [Crossref] [PubMed]
- Copetti R, Copetti P, Reissig A. Clinical integrated ultrasound of the thorax including causes of shock in nontraumatic critically ill patients. A practical approch. Ultrasound Med Biol 2012;38:349-59. [Crossref] [PubMed]
- Picano E, Pellikka PA. Ultrasound of extravascular lung water: a new standard for pulmonary congestion. European Heart Journal 2016;37:2097-104. [Crossref] [PubMed]
- Phillips CR, Chesnutt MS, Smith SM. Extravascular lung water in sepsis associated acute respiratory distress syndrome: indexing with predicted body weight improves correlation with severity of illness and survival. Crit Care Med 2008;36:69-73. [Crossref] [PubMed]
- Rhodes A, Evans LE, Alhazzani W, et al. Surviving sepsis campaign: international guidelines for management of sepsis and septic shock: 2016. Intensive care Med 2017;43:304-77. [Crossref] [PubMed]
- Goligher EC, Leis JA, Fowler RA, et al. Utility and safety of draining pleural effusions in mechanically ventilated patients: a systematic review and meta-analysis. Critical Care 2011;15:R46. [Crossref] [PubMed]
- Cartaxo AM, Vargas FS, Salge JM, et al. Improvements in the 6-min walk test and spirometry following thoracentesis for symptomatic pleural effusions. Chest 2011;139:1424-9. [Crossref] [PubMed]
- Baldi G, Gargani L, Abramo A, et al. Lung water assessment by lung ultrasonography in intensive care: a pilot study. Intensive Care Med 2013;39:74-84. [Crossref] [PubMed]
- Lichtenstein D, Goldstein I, Mourgeon E, et al. Comparative diagnostic performances of auscultation, chest radiography, and lung ultrasonography in acute respiratory distress syndrome. Anesthesiology 2004;100:9-15. [Crossref] [PubMed]
- Blackmore CC, Black WC, Dallas RV, et al. Pleural fluid volume estimation: a chest radiograph prediction rule. Acad Radiol 1996;3:103-9. [Crossref] [PubMed]
- Kocijancic I, Vidmar K, Ivanovi-Herceg Z. Chest sonography versus lateral decubitus radiography in the diagnosis of small pleural effusions. J Clin Ultrasound 2003;31:69-74. [Crossref] [PubMed]
- Sajadieh H, Afzali F, Sajadieh V, et al. Ultrasound as an alternative to aspiration for determining the nature of pleural effusion, especially in older people. A Ann N Y Acad Sci 2004;1019:585-92. [Crossref] [PubMed]
- Yang PC, Luh KT, Chang DB, et al. Value of sonography in determining the nature of pleural effusion: analysis of 320 cases. AJR Am J Roentgenol 1992;159:29-33. [Crossref] [PubMed]
- Vignon P, Chastagner C, Berkane V, et al. Quantitative assessment of pleural effusion in critically ill patients by means of ultrasonography. Crit Care Med 2005;33:1757-63. [Crossref] [PubMed]
- Brogi E, Gargani L, Bignami E, et al. Thoracic ultrasound for pleural effusion in the intensive care unit: a narrative review from diagnosis to treatment. Crit Care 2017;21:325. [Crossref] [PubMed]
- Balik M, Plasil P, Waldauf P, et al. Ultrasound estimation of volume of pleural fluid in mechanically ventilated patients. Intensive Care Med 2006;32:318. [Crossref] [PubMed]
- Vetrugno L, Bove T. Lung ultrasound estimation of pleural effusion fluid and the importance of patient position. Ann Intensive Care 2018;8:125. [Crossref] [PubMed]
- Vetrugno L, Brogi E, Barbariol F, et al. A Message in the Bottle Anesthesiology 2018;128:677. [Crossref] [PubMed]
- Remérand F, Dellamonica J, Mao Z, et al. Multiplane ultrasound approach to quantify pleural effusion at the bedside. Intensive Care Med 2010;36:656-64. [Crossref] [PubMed]
- Usta E, Mustafi M, Ziemer G. Ultrasound estimation of volume of postoperative pleural effusion in cardiac surgery patients. Interact Cardiovasc Thorac Surg 2010;10:204-7. [Crossref] [PubMed]
- Havelock T, Teoh R, Laws D, et al. BTS Pleural Disease Guideline Group. Pleural procedures and thoracic ultrasound: British Thoracic Society Pleural Disease Guideline 2010. Thorax 2010;65 Suppl 2:ii61-76. [Crossref] [PubMed]
- Wrightson JM, Fysh E, Maskell NA, et al. Risk reduction in pleural procedures: sonography, simulation and supervision. Curr Opin Pulm Med 2010;16:340-50. [Crossref] [PubMed]
- Barnes TW, Morgenthaler TI, Olson EJ, et al. Sonographically guided thoracentesis and rate of pneumothorax. J Clin Ultrasound 2005;33:442-6. [Crossref] [PubMed]
- Vetrugno L, Guadagnin GM, Orso D, et al. An easier and safe affair, pleural drainage with ultrasound in critical patient: a technical note. Crit Ultrasound J 2018;10:18. [Crossref] [PubMed]
- Salamonsen M, Ellis S, Paul E, et al. Thoracic ultrasound demonstrates variable location of the intercostal artery. Respiration 2012;83:323-9. [Crossref] [PubMed]
- Hew M, Rahman N. Preventing intercostal vessel trauma: ultrasound to the rescue once more? Respirology 2013;18:891-2. [Crossref] [PubMed]
- Koyanagi T, Kawaharada N, Kurimoto Y, et al. Examination of intercostal arteries with transthoracic Doppler sonography. Echocardiography 2010;27:17-20. [Crossref] [PubMed]
- Hibbert RM, Atwell TD, Lekah A, et al. Safety of ultrasound-guided thoracentesis in patients with abnormal preprocedural coagulation parameters. Chest 2013;144:456-63. [Crossref] [PubMed]
- Volpicelli G. Sonographic diagnosis of pneumothorax. Intensive Care Med 2011;37:224-32. [Crossref] [PubMed]
- Zanobetti M, Scorpiniti M, Gigli C, et al. Point-of-care ultrasonography for evaluation of acute dyspnea in the emergency department. Chest 2017;151:1295-301. [Crossref] [PubMed]
- Szarpak L, Mateo RG, Marchese G, et al. Ultrasonography as a tool for prehospital recognition of tension pneumothorax. Am J Emerg Med 2016;34:1302-3. [Crossref] [PubMed]
- Bainbridge D, McConnell B, Royse C. A review of diagnostic accuracy and clinical impact from the focused use of perioperative ultrasound. Can J Anaesth 2018;65:371-80. [Crossref] [PubMed]
- Ding W, Shen Y, Yang J, et al. Diagnosis of pneumothorax by radiography and ultrasonography: a meta-analysis. Chest 2011;140:859-66. [Crossref] [PubMed]
- Alrajhi K, Woo MY, Vaillancourt C. Test characteristics of ultrasonography for the detection of pneumothorax. Chest 2012;141:703-8. [Crossref] [PubMed]
- Azad A, Juma SA, Bhatti JA, et al. Validity of ultrasonography to diagnosing pneumothorax: a critical appraisal of two meta-analyses. CJEM 2015;17:199-201. [Crossref] [PubMed]
- Alrajab S, Youssef AM, Akkus NI, et al. Pleural ultrasonography versus chest radiography for the diagnosis of pneumothorax: review of the literature and meta-analysis. Critical Care 2013;17:R208. [Crossref] [PubMed]
- Staub LJ, Biscaro RRM, Kaszubowski E, et al. Chest ultrasonography for the emergency diagnosis of traumatic pneumothorax and haemothorax: a systematic review and meta-analysis. Injury 2018;49:457-66. [Crossref] [PubMed]
- Ketelaars R, Gülpinar E, Roes T, et al. Which ultrasound transducer type is best for diagnosing pneumothorax? Crit Ultrasound J 2018;10:27. [Crossref] [PubMed]
- Moreno-Aguilar G, Lichtenstein D. Lung ultrasound in the critically ill (LUCI) and the lung point: a sign specific to pneumothorax which cannot be mimicked. Critical Care 2015;19:311. [Crossref] [PubMed]
- Volpicelli G, Boero E, Sverzellati N, et al. Semi-quantification of pneumothorax volume by lung ultrasound. Intensive Care Med 2014;40:1460-7. [Crossref] [PubMed]
- Steenvoorden TS, Hilderink B, Elbers PWG, et al. Lung point in the absence of pneumothorax. Intensive Care Med 2018;44:1329-30. [Crossref] [PubMed]
- Retief J, Chopra M. Pitfalls in the ultrasonographic diagnosis of pneumothorax. J Intensive Care Soc 2017;18:143-5. [Crossref] [PubMed]
- Aziz SG, Patel BB, Ie SR, et al. The lung point sign, not pathognomonic of a pneumothorax. Ultrasound Quarterly 2016;32:277-9. [Crossref] [PubMed]
- Volpicelli G, Boero E, Stefanone V, et al. Unusual new signs of pneumothorax at lung ultrasound. Crit Ultrasound J 2013;5:10. [Crossref] [PubMed]
- Karacabey S, Sanri E, Metin B, et al. Use of ultrasonography for differentiation between bullae and pneumothorax. Emerg Radiol 2019;26:15-9. [Crossref] [PubMed]
- Montanari G, Orso D, Guglielmo N, et al. Comparison of different methods of size classification of primary spontaneous pneumothorax. Am J Emerg Med 2018;36:327-8. [Crossref] [PubMed]
- Elliot J. An Unexpected Light (Travels in Afghanistan). New York: Picador USA, 1999.
Cite this article as: Vetrugno L, Bove T, Guadagnin GM, Orso D, Brussa A, Volpicelli G. Advances in lung ultrasound in critically ill patients. J Emerg Crit Care Med 2019;3:32.