Monitoring the post surgery inflammatory host response
Introduction
Surgical procedures elicit a physiological inflammatory host response that comprises of an intricate mix of metabolic, immunological, hemodynamic and hormonal signals which are designed to be protective and create a physiological milieu that is conducive to recovery. This response is geared to ensure energy production and restoring cardiovascular homeostasis to promote tissue repair, wound healing and ultimately ensure patient wellbeing. Occasionally the inflammatory response is unable to confer its protective role and the patient may develop postoperative complications or even succumb to the effects of an amplified or weakened inflammatory response. The risk for this arises in scenarios where there is an interplay of various factors including patient factors, intraoperative complications, the nature and duration of the surgery as well as anaesthetic agents that may adversely influence and alter these complex pathways.
Understanding the inflammatory response to surgery and in particular being able to track the immune response in the post surgical period is advantageous as it poses to clinicians the opportunity to adjudge the patient’s response to the surgical insult, course of recovery, detection of complications including infections and the timely management thereof. This review explores the inflammatory response to surgery and the utility of currently available as well as promising biomarkers in this regard.
Inflammatory response to surgery
Metabolic and hormonal response
The metabolic, hemodynamic and hormonal response follows the activation of the hypothalamic-pituitary-adrenal (HPA) axis. It is characterized by the increased secretion of catecholamines, growth hormone, arginine vasopressin and cortisol as well a state of insulin resistance and an increase in gluconeogenesis, glycogenolysis and lipolysis. These neurological-endocrine pathways interact with the immune system and the net effect is to mobilise energy sources and maintain physiological homeostasis. The scale of tissue injury or surgical trauma influences the magnitude of the hormonal and metabolic response.
Immune response
During surgery, the occurrence of cellular disruption from tissue injury, haemorrhage or ischemia-reperfusion injury results in the activation of the innate and adaptive responses which are respectively pro-inflammatory and anti-inflammatory in nature. The former is defensive and destroys harmful molecules, whilst the latter focuses on promoting healing by containing the inflammatory process locally. Usually this local response suffices and results in the restoration of physiological homeostasis; however in a subset of patients a dysregulated response that is imbalanced ensues. This is characterised by excessive cytokine release, which manifests as a systemic inflammatory response syndrome (SIRS), and may culminate with either organ dysfunction or alternatively an increased predisposition for new or repeat infections.
Innate response
The innate response is typically characterised by phagocytosis as well as the activation of complement and natural killer cells. Following tissue injury, mediators such as oxygen reactive species, cytokines and nitric oxide are released, leading to a local response that is typified by the accumulation of macrophages, polymorphonuclear leucocytes and lymphocytes at the site of injury (1,2). Molecules such as high-mobility group B1(HMGB1), mitochondrial DNA, glycosaminoglycans, heat shock protein (HSP), adenosine triphosphate (ATP), protein S100 and uric acid are also released following tissue injury and they are collectively referred to as damage associated molecular patterns (DAMPs) (3,4). Toll like receptors (TLRs) such as TLR-2, TLR-4 and TLR-9 attract these DAMPs and the combined complex triggers a cascade of events which includes activation of the innate immune system (as well as the acquired/adaptive immune system). The innate system activation leads to release of cytokines such as tumour necrosis factor-α (TNF-α), interleukin-1β (IL-1β), IL-6 and IL-8 through nuclear factor kappa β (NFKβ) cell expression (5). Monocytes and macrophages in particular as well as lymphocytes, mast cells, endothelial cells and fibroblasts are responsible for the release of cytokines at the site of tissue injury (6,7).
These cytokines are the key drivers of the immune response and are detectable very soon following injury. Their roles include the regulation of immune function, prevention of cellular damage and the promotion of cellular repair. IL-6 in particular is instrumental in the release of complement, C-reactive protein (CRP) and procalcitonin (PCT) (8). TNF-α and IL-6 stimulate the production of CRP and PCT, whilst interferon-γ (INF-γ) suppresses the production of PCT. It has been demonstrated that the magnitude of IL-6 correlates with the severity of injury, duration of the surgery as well as the complications that ensue (9,10).
The cytokines that are produced may confer pro-inflammatory (IL-1, IL-6, TNF) or alternatively anti-inflammatory (IL-10) activity in that they augment or attenuate the SIRS response respectively. The former is instrumental in driving organ dysfunction whilst the latter renders the patient susceptible to infections. Until recently, it was believed that the pro-inflammatory response and the anti-inflammatory response were bimodal in nature. However, it is now accepted that they often occur almost simultaneously. When there is an imbalance in the pro-inflammatory and anti-inflammatory response or if either are left unchecked, the patient mounts a massive cytokine storm which manifests as dysregulated inflammatory response (11). The cytokine storm that ensues can drive multi-organ dysfunction (predominantly pro-inflammatory response) or lead to an increased susceptibility to infections (preponderance of anti-inflammatory response). An amplified pro-inflammatory response is likely in situations of extensive tissue injury, which may occur with damage control surgery, prolonged duration of surgery, intraoperative complications or long cardiopulmonary bypass (CPB) duration. Patients with immunosuppression may be at risk of a profound anti-inflammatory response. A dysregulated response thus sets the scene for potentially poor clinical outcomes.
Adaptive immunity
Activation of the acquired/adaptive immunity consisting of T helper (TH) lymphocytes follows the stress of surgery. TH1 lymphocytes regulate cell mediated immunity (CMI) through monocyte activation, whilst TH2 lymphocytes are responsible for antibody production. IL-12 produced from the activated monocytes of the innate response, induces TH1 and TH2 cells. In turn TH cells also drive the secretion of IL-10 and the production of cytokines. TH1 cells thus promote pro-inflammatory activity and cellular immunity whilst TH2 cells promote anti-inflammatory activity (8,12).
It has been observed that surgery results in an imbalance of TH1 and TH2 lymphocytes in favour of TH2 cells. Elevated IL-10 levels are also encountered as a result of the innate response (13-15). The TH1 and TH2 cell imbalance is attributed to elevated prostaglandins being due to an increase in IL-10 as well as through glucocorticoid mediation consequent to the stress related activation of the HPA axis and the subsequent increase in cortisol and catecholamines. As such, CMI is often suppressed following surgery and patients are predisposed to the development of postoperative infections and complications (9,10,14,16).
The immune response to an infection follows a similar process except that the trigger is a component of the microorganism, which is recognised by the host’s TLRs as a pathogen-associated molecular pattern (PAMP). Figure 1 illustrates that both tissue injury as well as pathogens can activate the inflammatory response through the release of DAMPs or PAMPs, which may culminate with either clinical recovery, organ dysfunction or with an increased propensity for infections and that the patient may succumb to the injury or infection if the inflammatory response remains imbalanced.
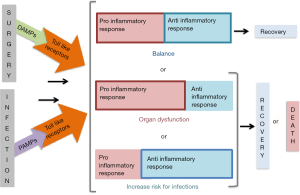
Monitoring the immuno-inflammatory response post-surgery
Taking into account the risk of postoperative infections and surgery specific complications (ischemia, haemorrhage, anastomotic leaks), mapping and monitoring the immune-inflammatory response to surgery poses an attractive opportunity to timeously identify such complications. This is however limited by the complexity of the multiple interdependent pathways, the overlap in the inflammatory pathways which occurs with both infectious and non-infectious complications as well as a multitude of extraneous factors which may alter the immune response such as patient comorbidities, pre-surgical immune status, age, mode of anaesthesia, anaesthetic agent, duration of the surgical procedure and the occurrence of intraoperative complications. In the absence of rapid and reliable diagnostic tools to permit early identification of infectious and non-infectious complications, there is a huge reliance on the use of biomarkers to map the immune response. As such, biomarkers often serve as adjunctive tools to guide the management of postoperative patients. From a biomarker use perspective, it is now appreciated that due to the dynamic fluxes in the immune response and the heterogeneous nature of patients, a “one size fits all” approach is not useful; instead an individualised approach is deemed to yield an enhanced interpretation of the clinical scenarios that we deal with.
Biomarkers
To date short of 200 biomarkers have been evaluated or are currently being investigated for their potential role in this domain but their poor discriminatory performance in heterogeneous patient populations has precluded the use of the vast majority of these biomarkers. Candidate biomarkers include cytokines, acute phase proteins, coagulation cascade markers, neutrophil activation factors as well as other specific markers of the immune response. The current focus is now steering towards the use of combination biomarkers in order to improve their discriminatory power (17,18). Further the utility of genomic and proteomic technologies are also being investigated (19,20).
Cytokines
These chemically active molecules play a central role in the inflammatory response, which renders them attractive as monitoring tools, which could be used to accurately determine the precise phase of the inflammatory response based on the ratio of the various cytokines at a given point in time. Their routine use is however precluded by their ultra-short half-lives (which would necessitate multiple tests with rapid turn-around times for meaningful management decisions), the presence of blocking antibodies, a lack of access to them, a lack of decision-making algorithms and the cost of the tests. As such they are currently utilised mainly in research settings.
IL-6 has been studied the most amongst the cytokines. It is detectable 2 hours post-surgery and peaks 12–24 hours post-surgery and declines to normal levels within 48–72 hours in the absence of complications (21). Its ultra-short half-life precludes its routine use.
Although CRP and PCT are both induced by cytokines they each have their own strengths and weaknesses. They play a useful role in the postoperative setting and this is elaborated upon below.
CRP
CRP is a non-specific inflammatory marker produced by the hepatocytes. It exhibits a half-life of 19 hours and elevated levels are first detectable 6 hours post insult or surgery and it peaks at 48–72 hours post-surgery (22). CRP activates complement and has numerous functions in the inflammatory response including cytokine activation and elimination of target cells (23,24). In the absence of complications, CRP levels start declining from day 3 to 4 post surgery when the stimulus for its production (DAMPs induced cytokine production) ceases and it may take up to a week for CRP levels to return to baseline levels (<3 mg/L) (25-27). It exhibits a slow decay, which limits its utility in the immediate postoperative period. The magnitude of the upswing in the CRP level is believed to be proportional with the scale of the inflammatory response. Elevated CRP levels occur with conditions that lead to an inflammatory response and this includes infections (bacterial, fungal and viral), surgery, trauma, malignancy, CPB, pancreatitis, necrosis, ischemia, burns and massive transfusion. Hepatic dysfunction impedes CRP production.
PCT
PCT is produced in the thyroid glands, lungs and intestinal tissue. It has a short half-life of 24 hours and in healthy individuals plasma levels are below 0.15 ng/mL. Infections and non-infectious insults (including surgery and any cause of a SIRS) lead to an increase in PCT and the source of production broadens to include several other organs. Hence PCT levels can increase significantly. PCT production is highly specific for bacterial infections. PCT is mainly induced by IL-6 and it is detectable within 3–6 hours of the injury or insult. The greater the scale of the inflammatory response, the greater the magnitude of the PCT that is subsequently produced. Any patient who mounts a non-infectious SIRS response should manifest with an increase in PCT production. As such patients with burns, pancreatitis, massive transfusion, myocardial infarction or post surgery will exhibit an elevated PCT. Clec’h et al. demonstrated that the baseline median PCT among medical patients was significantly lower than that of post surgical patients which supports the notion of moving away from focusing on absolute values and reference ranges of PCT in order to interpret the inflammatory response (28).
PCT has been studied extensively in terms of its potential role in the diagnosis of bacterial and fungal infections, its reduction on duration of antimicrobial therapy, its ability to detect postoperative complications and in prognosticating patient outcome. Certainly, all the trials are not in agreement in terms of their findings regarding its utility, but a common thread has emerged in that the use of PCT kinetics provides a useful adjunctive tool in terms of detecting complications and curtailing the duration of antibiotic use (29-36). The following factors help guide the individualised approach to interpreting PCT:
- Patients with a non-infectious cause for SIRS such as burns, polytrauma, a major elective surgical procedure or a massive blood transfusion, will exhibit an elevated PCT level. The level of elevation corresponds to the magnitude of the SIRS response or cytokine storm. However, as the underlying cause for the SIRS response resolves, there should be a proportional decline in the inducing DAMPs levels and therefore the PCT should steadily decline towards normal levels. As such if the PCT kinetics does not show a decline in such patients there should be a concern of either a new infection or on-going primary pathology.
- It has been demonstrated that bacterial infections elicit a massive cytokine storm and as such PCT elevations are greater with bacterial infections compared to that following elective surgical procedures. Clec’h et al. demonstrated that patients with septic shock display a 10-fold higher median PCT concentration than patients with sterile shock (37).
- INF-γ, the predominant cytokine that is produced in viral infections, suppresses PCT production and as such patients with pure viral infections will exhibit a normal PCT level.
- Post surgery the magnitude of PCT elevation depends on the extent of the induced inflammatory response as well as the site of the surgery. Typically, PCT elevations are greatest with abdominal and retroperitoneal surgery (26,27,38). Thoracotomies and other surgical site incisions evoke a smaller PCT induction than do laparotomies. Postoperative PCT elevations of up to 9 ng/mL have been observed. Postoperatively, the PCT has been observed to peak within 24 hours and thereafter decline steadily daily, to normal levels provided there are no postoperative complications including infections (26,27). Any PCT level greater than 10 ng/mL post surgery in the non-transplant setting should generally be regarded as abnormal. A PCT that plateaus or does not decline 24 hours after surgery is also considered to be abnormal and may allude to either a postoperative complication, new infection or on-going infection (in the case of preoperative sepsis).
- Prolonged CPB duration rather than CPB itself induces PCT production.
- Transplantation surgery has been shown to induce PCT levels above 10 ng/mL (25).
- In situations of repeat infections, the peak PCT level for each subsequent infection tends to be less than the peak PCT of the preceding infection (39,40). This may be attributed to a lesser cytokine storm occurring with subsequent infections; possibly due to the development of a weakened immune response over time. This phenomenon has been demonstrated in patients with secondary peritonitis (39). As such to effectively track the occurrence of repeat infections, the PCT kinetics becomes more important than an individual PCT value.
In the surgical critical care patient population, the aforementioned makes a strong case for the use of PCT trends or kinetics rather than the use of reference ranges or individual values. Further, PCT as per other biomarkers should always be evaluated and interpreted within the context of the clinical scenario while taking into account its limitations.
White cell count (WCC)
Changes in WCCs are not well described in the literature largely due to their poor specificity in terms of detecting postoperative complications.
Future directions
Currently most clinicians rely upon CRP and PCT to adjudge the inflammatory response and guide therapy. Biomarkers that are being explored in this domain include but are not limited to adrenomedullin, soluble triggering receptor expressed on myeloid cells-1 (sTREM-1), pro-adenomedullin1, CD-64 and sCD-14. It has been suggested that a panel comprising of calprotectin M-1, IL-6 and PCT may be more specific and provide a better reflection of the interaction of the different components and determine the phase of the inflammatory response at specific points in time—chemotactic, initial hours and then a more generalised response respectively—to detect postoperative infections before the onset of clinical manifestations (41,42).
Markers of immunosuppression are considered to be attractive to monitor, in order to adjudge the state of the adaptive immune response as immunosuppressed patients may benefit from immunostimulatory therapy. To this extent, the use of flow cytometry to ascertain the extent of immunosuppression is being explored. Parameters such as CD10/CD16 expression, human leukocyte antigen-DR (HLA-DR) expression on monocytes, CD4 and lymphocyte counts comprise the markers that are being explored (43).
We need to accept that a single marker will probably never be able to reflect the full spectrum of changes and the rapidity with which they occur. Further, the overlap in the response to both infections and non-infectious insults is a huge limiting factor when it comes to being able to tease out infections from non-infectious complications. As such, the multipanel biomarker route is inevitable in the future.
Role of PCT and CRP in the postoperative setting
The use of biomarker kinetics is key in the postoperative period. Whilst all the studies may not support the utility of PCT and CRP, there is a body of evidence to supports that PCT and CRP kinetics are helpful in the identification of postoperative complications. CRP however tends to yield this signal later than PCT as it is a biomarker that reacts more slowly than PCT. It is recommended that both be monitored as neither are perfect, as per their limitations which are described below. PCT kinetics are especially useful as they play a more specific role in the detection of bacterial infections, provide guidance on when to stop antibiotics and thus reduce antibiotic exposure, provide a cue to consider the possibility of a fungal infection and also offer an impression of the severity of the injury or infection. This is discussed in detail below and a practical approach is depicted in Figure 2. Box 1 summarises key messages pertaining to the role of CRP and PCT in the postoperative period.
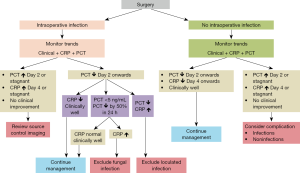
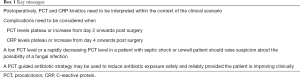
Full table
Detecting post-operative infections
Infections are difficult to detect. They are not always clinically apparent or amenable for detection by imaging. Blood cultures have a poor yield with turn-around times that usually exceed 48 hours. Clinical and biological markers of inflammation such as hypotension or aberrations in temperature, respiratory rate, heart rate and WCC confer poor specificity for infections. Further, sepsis follows a very complex inflammatory response that is almost identical to that exhibited by non-infectious causes of tissue damage. Thus inflammatory changes due to infections are indistinguishable from non-infectious SIRS response. Taking into account that time to source control impacts on outcome, CRP and PCT are relied upon to better inform us about the clinical situations that we manage.
Whilst the CRP and PCT response to surgery is similar, the main difference between them is that CRP and PCT peak at 48–72 hours and 24 hours respectively. Thus, CRP kinetics can really only be used from 72 hours postoperatively onwards. Postoperative complications might thus be detected much sooner with PCT use. Another drawback of CRP relates to it reaching a plateau quickly following an insult irrespective of the severity of the insult, whilst the PCT response has been observed to be proportional to the severity of the infection (44). The rationale for monitoring PCT and CRP relates to both biomarkers having their own inherent limitations. PCT may not be elevated in loculated or deep seated infections and in such cases a stagnant CRP should alert the clinician to consider further investigations such as imaging especially if the patient is not improving clinically.
Several studies have reported that PCT is useful in the detection of bacterial infections and it has also been demonstrated to be more sensitive and specific than CRP, WCC and IL-6 in detecting infections (45,46). These are studies in mixed medico-surgical populations. There are also a few studies that have contradicted its utility in detecting infections (47-52). This may be attributed to the use of absolute values rather than PCT kinetics.
In critically ill patients or post-operative patients the inevitable occurrence of non-infectious SIRS precludes the use of the normal reference ranges for PCT in order to adjudge the presence or absence of an infection. This might explain why certain studies have been negative regarding PCT utility in critically ill patients.
A recent study by Trásy et al. supports the notion of monitoring PCT kinetics to improve the discriminatory power of sepsis detection (53). They determined the delta-PCT (PCT level from preceding day subtracted from PCT level on day of suspected infection) and found that patients with an infection exhibited a significantly higher delta PCT than those without an infection. A delta-PCT value of 0.76 yielded the best discrimination for infection whilst absolute values or changes in WCC and CRP were not deemed useful. Similarly, Tsangaris et al. demonstrated that a twofold increase in PCT within a 24-hour period together with fever was useful to detect infections in an intensive care unit (ICU) population (54). This study highlights the importance of monitoring kinetics rather than absolute values as a twofold increase was also observed in patients with very low PCT baseline levels (in the range 0.1 and 0.75 ng/mL).
Among pancreaticoduodenectomy patients, both CRP and PCT appear to be useful in predicting infectious complications (55). A recent systematic review supports the use of PCT to predict intra-abdominal infections post colorectal surgery (35). An earlier meta-analysis did not support this and it might be attributed to the use of absolute values in the studies rather than kinetics (56). PCT has also been reported to be useful in the detection of infections post lung and cardiac surgery (30,31,57).
Postoperatively a patient developing a new infection would have both DAMPs and PAMPs driven PCT production. Infections should thus be considered in situations where the patient is clinically unwell and the PCT as well as CRP is static or not declining.
Post transplantation, PCT induction is not suppressed whilst CRP production may be suppressed with the administration of immunosuppressive therapy. A normal decay of PCT post transplant would help differentiate rejection from an infection.
Gram negative infections including multidrug resistant gram negative pathogens have been reported to provoke a greater induction of PCT than do gram positive pathogens (58,59). However, it is not advised to use the magnitude of the PCT to inform empiric antibiotic therapy choices, particularly in critically ill patients who also often exhibit a state of immunoparesis.
The absence of a postoperative CRP rise following surgery may help to exclude an infection (60). However, this would generally entail several days of CRP monitoring to permit the normal course of CRP decline to occur. CRP kinetics has been reported to be useful to detect infectious complications postoperatively by observing the trend over at least 4–7 days post surgery (61-63).
Amongst the available biomarkers PCT has been found to confer the best discriminatory ability for bacterial infections.
Monitoring response to antimicrobial therapy or intra-operative source control
Novotny et al., demonstrated in a cohort of 104 patients with septic peritonitis that a day 1: day 2 PCT ratio greater than 1.03 is useful to tease out successful cases of source control [area under curve (AUC), 0.83] (64). A few other studies have also demonstrated the usefulness of PCT kinetics in detecting the occurrence of new sepsis or on going sepsis postoperatively (32-34). A study that found PCT to be of no value in monitoring response to therapy in abdominal infections did however require that the PCT fall to <0.05 ng/mL (65).
The Early Procalcitonin Kinetics (EProK) study demonstrated PCT kinetics to be useful in adjudging response to antibiotic therapy. PCT was measured 8 hourly in the first 24 hours post antibiotic initiation and patients with inappropriate antibiotic therapy demonstrated a PCT that increased through the first 24 hours whilst the appropriate therapy group demonstrated a fall in the PCT between 16 and 24 hours post antibiotic initiation (36). Clearance of CRP and PCT together with clinical improvement is a reassuring feature in patient management (66,67).
Fungal infections
PCT levels are reported to be typically low (<5.5 ng/mL) with a median value of 0.6 ng/mL in fungal sepsis (68,69). This may be due to suppression by INF-γ. As such if a patient has a low PCT level and is clinically unwell and an infection is suspected, one should be considering the possibility of a fungal infection. Patients who have a bacterial infection may also develop a fungal infection and in such situations one would expect the PCT level to suddenly decline by more than 50% within a 24 hour period yet the patient would remain clinically unimproved or be more ill. In a patient with septic shock who is deteriorating and the PCT level is <5 mg/mL or declining rapidly, a fungal infection ought to be considered.
Detecting non-infectious complications
The use of CRP and or PCT levels post abdominal surgery have yielded conflicting results in terms of detecting postoperative complications and this may be due to population heterogeneity, small cohorts or the use of absolute values rather than kinetics (70-76). However, the majority of studies do suggest the utility of CRP and or PCT in detecting postoperative complications (72-76). It also appears that the PCT may be used earlier than the CRP to identify complications (73,75).
Post-abdominal surgery, the detection of anastomotic leaks is crucial to avoid progression to fulminant sepsis. It has been reported that the use of CRP and PCT is useful in this setting (75). Post cardiac surgery, presepsin and PCT have been found to behave similarly in terms of identifying postoperative complications on day 2 in a cohort of 122 patients (77). In a recent study with a cohort of 72 patients post liver resection, PCT was found to be superior to WCC and CRP in terms of detecting postoperative complications and this was possible within 2 days of surgery (76).
Monocytic HLA-DR expression appears to be more promising in comparison to WCC, CRP and PCT to predict anastomotic leaks post colorectal surgery (78). This was useful from postoperative days 3 and 4 onwards.
Duration of antibiotic therapy
A PCT guided strategy has been shown to safely reduce the duration of antibiotic exposure in several trials (29,79-83). These studies include postoperative patients as well as patients with intra-abdominal infections and have not demonstrated an increased mortality with such a strategy. The PCT guided strategy entails stopping antibiotic therapy provided the patient is clinically improving and there is a decline in the PCT by 80% compared to the peak PCT response for that infection. Surgical source control would be necessary in cases of surgical infections. Shehabi et al. recently demonstrated no impact on duration of antibiotic exposure with a PCT guided strategy but this trial has been criticised for its overly ambitious objective of reducing antibiotic exposure (a 25% reduction which essentially translates to antibiotic discontinuation within 96 hours of initiation) (84). Earlier studies that showed benefit were conducted during the era of prolonged periods of antibiotic administration and have also been appropriately criticised in terms of changes in our current antibiotic prescription practices. A recent study from the Netherlands not only reaffirms but also provides very good data that even during the era of reduced antibiotic exposure, a PCT guided strategy can significantly curtail the duration of antibiotic exposure with added benefits (29). PCT guided strategies to reduce antibiotic exposure in patients with abdominal infections have been described (80-83). Currently Infectious Diseases Society of America (IDSA) and the Surviving Sepsis Campaign guidelines suggest the use of PCT for antibiotic cessation (85,86).
Specific considerations
Renal dysfunction
Patients with renal dysfunction or who are receiving intermittent renal replacement therapy have been shown to exhibit higher basal levels of PCT as the half-life does increase with renal dysfunction (87). The decline rate of PCT is however unaltered and kinetics should be more useful than the actual individual values (88-90). It is also recommended that the PCT measurements be deferred for at least 3 hours post dialysis.
Neutropenia/immunosuppression
PCT has been shown to be superior to CRP and IL-8 in detecting infections in neutropenic patients (91). As actual values may be blunted, trends may well be more useful in this population. Immunosuppression does reduce the induction of PCT. However once again the kinetics of the PCT should be used rather than absolute values.
Prognosis
The magnitude of the peak PCT response as well as the rates of clearance of PCT and CRP have been found to reflect the severity of disease and predicting outcome in many of the reported studies (39,47,61,62,92,93). IL-6, TNF-α and PCT have been demonstrated to be good prognosticators of mortality (41). Pre transplantation PCT levels have been suggested to prognosticate transplant success (25).
Conclusions
The heterogeneity of the patients we manage together with the complexities and the overlap that surround the PAMPs and DAMPs induced inflammatory response necessitates that we try our best to map this process as it evolves and culminates with recovery, organ dysfunction or death. The biomarkers at our disposal are far from adequate but there is evidence to support the use of PCT and CRP kinetics—provided it is interpreted against the clinical backdrop—to inform our decision-making and guide our management.
Acknowledgments
None.
Footnote
Conflicts of Interest: Paruk F has served on the speaker bureau for Fresenius Kabi, Pfizer, Cytosorbents and MSD. The other author has no conflicts of interest to declare.
Ethical Statement: The authors are accountable for all aspects of the work in ensuring that questions related to the accuracy or integrity of any part of the work are appropriately investigated and resolved.
References
- Hsing CH, Wang JJ. Clinical implication of perioperative inflammatory cytokine alteration. Acta Anaesthesiol Taiwan 2015;53:23-8. [Crossref] [PubMed]
- Toft P, Tønnesen E, Helbo-Hansen HS, et al. Redistribution of granulocytes in patients after major surgical stress. APMIS 1994;102:43-8. [Crossref] [PubMed]
- Hotchkiss RS, Moldawer LL. Parallels between cancer and infectious disease. N Engl J Med 2014;371:380-3. [Crossref] [PubMed]
- Hotchkiss RS, Moldawer LL, Opal SM, et al. Sepsis and septic shock. Nat Rev Dis Primers 2016;2:16045. [Crossref] [PubMed]
- Akira S, Takeda K. Toll-like receptor signalling. Nat Rev Immunol 2004;4:499-511. [Crossref] [PubMed]
- Spangler JB, Moraga I, Mendoza JL, et al. Insights into cytokine-receptor interactions from cytokine engineering. Annu Rev Immunol 2015;33:139-67. [Crossref] [PubMed]
- Hsing CH, Li HH, Hsu YH, et al. The distribution of interleukin-19 in healthy and neoplastic tissue. Cytokine 2008;44:221-8. [Crossref] [PubMed]
- Toft P, Tonnesen E. The systemic inflammatory response to anaesthesia and surgery. Curr Anaesth Crit Care 2008;19:349-53. [Crossref]
- Shenkin A, Fraser WD, Series J, et al. The serum interleukin 6 response to elective surgery. Lymphokine Res 1989;8:123-7. [PubMed]
- Mokart D, Capo C, Blache JL, et al. Early postoperative compensatory anti-inflammatory response syndrome is associated with septic complications after major surgical trauma in patients with cancer. Br J Surg 2002;89:1450-6. [Crossref] [PubMed]
- Cavaillon JM, Adib-Conquy M. Bench-to-bedside review: endotoxin tolerance as a model of leukocyte reprogramming in sepsis. Crit Care 2006;10:233. [Crossref] [PubMed]
- Marik PE, Flemmer M. The immune response to surgery and trauma: implications for treatment. J Trauma Acute Care Surg 2012;73:801-8. [Crossref] [PubMed]
- Roumen RM, Hendriks T, van der Ven-Jongekrijg J, et al. Cytokine patterns in patients after major vascular surgery, hemorrhagic shock, and severe blunt trauma. Relation with subsequent adult respiratory distress syndrome and multiple organ failure. Ann Surg 1993;218:769-76. [Crossref] [PubMed]
- Decker D, Schondorf M, Bidlingmaier F, et al. Surgical stress induces a shift in the type-1/type-2 T-helper cell balance, suggesting down-regulation of cell-mediated and up-regulation of antibody-mediated immunity commensurate to the trauma. Surgery 1996;119:316-25. [Crossref] [PubMed]
- O'Sullivan ST, Lederer JA, Horgan AF, et al. Major injury leads to predominance of the T helper-2 lymphocyte phenotype and diminished interleukin-12 production associated with decreased resistance to infection. Ann Surg 1995;222:482-90; discussion 490-2. [Crossref] [PubMed]
- Faist E, Kupper TS, Baker CC, et al. Depression of cellular immunity after major injury. Its association with posttraumatic complications and its reversal with immunomodulation. Arch Surg 1986;121:1000-5. [Crossref] [PubMed]
- Kofoed K, Andersen O, Kronborg G, et al. Use of plasma C-reactive protein, procalcitonin, neutrophils, macrophage migration inhibitory factor, soluble urokinase-type plasminogen activator receptor, and soluble triggering receptor expressed on myeloid cells-1 in combination to diagnose infections: a prospective study. Crit Care 2007;11:R38. [Crossref] [PubMed]
- Gibot S, Béné MC, Noel R, et al. Combination biomarkers to diagnose sepsis in the critically ill patient. Am J Respir Crit Care Med 2012;186:65-71. [Crossref] [PubMed]
- Pena OM, Hancock DG, Lyle NH, et al. An endotoxin tolerance signature predicts sepsis and organ dysfunction at initial clinical presentation. EBioMedicine 2014;1:64-71. [Crossref] [PubMed]
- Langley RJ, Tipper JL, Bruse S, et al. Integrative "omic" analysis of experimental bacteremia identifies a metabolic signature that distinguishes human sepsis from systemic inflammatory response syndromes. Am J Respir Crit Care Med 2014;190:445-55. [Crossref] [PubMed]
- Watt DG, Horgan PG, McMillan DC. Routine clinical markers of the magnitude of the systemic inflammatory response after elective operation: a systematic review. Surgery 2015;157:362-80. [Crossref] [PubMed]
- Colley CM, Fleck A, Goode AW, et al. Early time course of the acute phase protein response in man. J Clin Pathol 1983;36:203-7. [Crossref] [PubMed]
- Ballou SP, Lozanski G. Induction of inflammatory cytokine release from cultured human monocytes by C-reactive protein. Cytokine 1992;4:361-8. [Crossref] [PubMed]
- Volanakis JE. Acute phase proteins in rheumatic disease. In: Koopman WJ. editor. Arthritis and allied conditions: a textbook of rheumatology. 13th ed. Baltimore: Williams & Wilkins, 1997:505-14.
- Meisner M. Procalcitonin-biochemistry and clinical diagnosis. 1st ed. Bremen: Uni-Med Verlag Ag, 2010.
- Lindberg M, Hole A, Johnsen H, et al. Reference intervals for procalcitonin and C-reactive protein after major abdominal surgery. Scand J Clin Lab Invest 2002;62:189-94. [Crossref] [PubMed]
- Meisner M, Tschaikowsky K, Hutzler A, et al. Postoperative plasma concentrations of procalcitonin after different types of surgery. Intensive Care Med 1998;24:680-4. [Crossref] [PubMed]
- Clec'h C, Fosse JP, Karoubi P, et al. Differential diagnostic value of procalcitonin in surgical and medical patients with septic shock. Crit Care Med 2006;34:102-7. [Crossref] [PubMed]
- de Jong E, van Oers JA, Beishuizen A, et al. Efficacy and safety of procalcitonin guidance in reducing the duration of antibiotic treatment in critically ill patients: a randomised, controlled, open-label trial. Lancet Infect Dis 2016;16:819-27. [Crossref] [PubMed]
- Suberviola B, Rellan L, Riera J, et al. Role of biomarkers in early infectious complications after lung transplantation. PLoS One 2017;12:e0180202. [Crossref] [PubMed]
- Heredia-Rodríguez M, Bustamante-Munguira J, Lorenzo M, et al. Procalcitonin and white blood cells, combined predictors of infection in cardiac surgery patients. J Surg Res 2017;212:187-94. [Crossref] [PubMed]
- Tschaikowsky K, Hedwig-Geissing M, Braun GG, et al. Predictive value of procalcitonin, interleukin-6, and C-reactive protein for survival in postoperative patients with severe sepsis. J Crit Care 2011;26:54-64. [Crossref] [PubMed]
- Azevedo JR, Torres OJ, Czeczko NG, et al. Procalcitonin as a prognostic biomarker of severe sepsis and septic shock. Rev Col Bras Cir 2012;39:456-61. [Crossref] [PubMed]
- Bezmarevic M, Mirkovic D, Soldatovic I, et al. Correlation between procalcitonin and intra-abdominal pressure and their role in prediction of the severity of acute pancreatitis. Pancreatology 2012;12:337-43. [Crossref] [PubMed]
- Tan WJ, Ng WQ, Sultana R, et al. Systematic review and meta-analysis of the use of serum procalcitonin levels to predict intra-abdominal infections after colorectal surgery. Int J Colorectal Dis 2018;33:171-80. [Crossref] [PubMed]
- Trásy D, Tánczos K, Németh M, et al. Early procalcitonin kinetics and appropriateness of empirical antimicrobial therapy in critically ill patients: a prospective observational study. J Crit Care 2016;34:50-5. [Crossref] [PubMed]
- Clec'h C, Ferriere F, Karoubi P, et al. Diagnostic and prognostic value of procalcitonin in patients with septic shock. Crit Care Med 2004;32:1166-9. [Crossref] [PubMed]
- Molter GP, Soltész S, Kottke R, et al. Procalcitonin plasma concentrations and systemic inflammatory response following different types of surgery. Anaesthesist 2003;52:210-7. [Crossref] [PubMed]
- Rau BM, Frigerio I, Büchler MW, et al. Evaluation of procalcitonin for predicting septic multiorgan failure and overall prognosis in secondary peritonitis: a prospective, international multicenter study. Arch Surg 2007;142:134-42. [Crossref] [PubMed]
- Charles PE, Tinel C, Barbar S, et al. Procalcitonin kinetics within the first days of sepsis: relationship with the appropriateness of antibiotic therapy and the outcome. Crit Care 2009;13:R38. [Crossref] [PubMed]
- Dolin HH, Papadimos TJ, Stepkowski S, et al. A novel combination of biomarkers to herald the onset of sepsis prior to the manifestation of symptoms. Shock 2018;49:364-70. [Crossref] [PubMed]
- Kibe S, Adams K, Barlow G. Diagnostic and prognostic biomarkers of sepsis in critical care. J Antimicrob Chemother 2011;66 Suppl 2:ii33-40. [Crossref] [PubMed]
- Monneret G, Venet F. Sepsis-induced immune alterations monitoring by flow cytometry as a promising tool for individualized therapy. Cytometry B Clin Cytom 2016;90:376-86. [Crossref] [PubMed]
- Meisner M, Tschaikowsky K, Palmaers T, et al. Comparison of procalcitonin (PCT) and C-reactive protein (CRP) plasma concentrations at different SOFA scores during the course of sepsis and MODS. Crit Care 1999;3:45-50. [Crossref] [PubMed]
- Müller B, Becker KL, Schächinger H, et al. Calcitonin precursors are reliable markers of sepsis in a medical intensive care unit. Crit Care Med 2000;28:977-83. [Crossref] [PubMed]
- Domínguez-Comesaña E, Estevez-Fernández SM, López-Gómez V, et al. Procalcitonin and C-reactive protein as early markers of postoperative intra-abdominal infection in patients operated on colorectal cancer. Int J Colorectal Dis 2017;32:1771-4. [Crossref] [PubMed]
- Reith HB, Mittelkötter U, Wagner R, et al. Procalcitonin (PCT) in patients with abdominal sepsis. Intensive Care Med 2000;26 Suppl 2:S165-9. [Crossref] [PubMed]
- Wanner GA, Keel M, Steckholzer U, et al. Relationship between procalcitonin plasma levels and severity of injury, sepsis, organ failure, and mortality in injured patients. Crit Care Med 2000;28:950-7. [Crossref] [PubMed]
- Ugarte H, Silva E, Mercan D, et al. Procalcitonin used as a marker of infection in the intensive care unit. Crit Care Med 1999;27:498-504. [Crossref] [PubMed]
- Ruokonen E, Ilkka L, Niskanen M, et al. Procalcitonin and neopterin as indicators of infection in critically ill patients. Acta Anaesthesiol Scand 2002;46:398-404. [Crossref] [PubMed]
- Reith HB, Mittelkötter U, Debus ES, et al. Procalcitonin in early detection of postoperative complications. Dig Surg 1998;15:260-5. [Crossref] [PubMed]
- Takakura Y, Hinoi T, Egi H, et al. Procalcitonin as a predictive marker for surgical site infection in elective colorectal cancer surgery. Langenbecks Arch Surg 2013;398:833-9. [Crossref] [PubMed]
- Trásy D, Tánczos K, Németh M, et al. Delta procalcitonin is a better indicator of infection than absolute procalcitonin values in critically ill patients: a prospective observational study. J Immunol Res 2016;2016:3530752.
- Tsangaris I, Plachouras D, Kavatha D, et al. Diagnostic and prognostic value of procalcitonin among febrile critically ill patients with prolonged ICU stay. BMC Infect Dis 2009;9:213. [Crossref] [PubMed]
- Giardino A, Spolverato G, Regi P, et al. C-reactive protein and procalcitonin as predictors of postoperative inflammatory complications after pancreatic surgery. J Gastrointest Surg 2016;20:1482-92. [Crossref] [PubMed]
- Cousin F, Ortega-Deballon P, Bourredjem A, et al. Diagnostic accuracy of procalcitonin and C-reactive protein for the early diagnosis of intra-abdominal infection after elective colorectal surgery: a meta-analysis. Ann Surg 2016;264:252-6. [Crossref] [PubMed]
- Baykut D, Schulte-Herbrüggen J, Krian A. The value of procalcitonin as an infection marker in cardiac surgery. Eur J Med Res 2000;5:530-6. [PubMed]
- Arora S, Singh P, Singh PM, et al. Procalcitonin levels in survivors and nonsurvivors of sepsis: systematic review and meta-analysis. Shock 2015;43:212-21. [Crossref] [PubMed]
- Cabral L, Afreixo V, Meireles R, et al. Evaluation of procalcitonin accuracy for the distinction between gram-negative and gram-positive bacterial sepsis in burn patients. J Burn Care Res 2019;40:112-9. [Crossref] [PubMed]
- Mustard RA Jr, Bohnen JM, Haseeb S, et al. C-reactive protein levels predict postoperative septic complications. Arch Surg 1987;122:69-73. [Crossref] [PubMed]
- Woeste G, Müller C, Bechstein WO, et al. Increased serum levels of C-reactive protein precede anastomotic leakage in colorectal surgery. World J Surg 2010;34:140-6. [Crossref] [PubMed]
- Welsch T, Müller SA, Ulrich A, et al. C-reactive protein as early predictor for infectious postoperative complications in rectal surgery. Int J Colorectal Dis 2007;22:1499-507. [Crossref] [PubMed]
- Cruickshank AM, Hansell DT, Burns HJ, et al. Effect of nutritional status on acute phase protein response to elective surgery. Br J Surg 1989;76:165-8. [Crossref] [PubMed]
- Novotny AR, Emmanuel K, Hueser N, et al. Procalcitonin ratio indicates successful surgical treatment of abdominal sepsis. Surgery 2009;145:20-6. [Crossref] [PubMed]
- Jung B, Molinari N, Nasri M, et al. Procalcitonin biomarker kinetics fails to predict treatment response in perioperative abdominal infection with septic shock. Crit Care 2013;17:R255. [Crossref] [PubMed]
- Karlsson S, Heikkinen M, Pettilä V, et al. Predictive value of procalcitonin decrease in patients with severe sepsis: a prospective observational study. Crit Care 2010;14:R205. [Crossref] [PubMed]
- Póvoa P, Teixeira-Pinto AM, Carneiro AH, et al. C-reactive protein, an early marker of community-acquired sepsis resolution: a multi-center prospective observational study. Crit Care 2011;15:R169. [Crossref] [PubMed]
- Charles PE, Dalle F, Aho S, et al. Serum procalcitonin measurement contribution to the early diagnosis of candidemia in critically ill patients. Intensive Care Med 2006;32:1577-83. [Crossref] [PubMed]
- Huber W, Schweigart U, Bottermann P. Failure of PCT to indicate severe fungal infection in two immunodeficient patients. Infection 1997;25:377-8. [Crossref] [PubMed]
- Muñoz JL, Alvarez MO, Cuquerella V, et al. Procalcitonin and C-reactive protein as early markers of anastomotic leak after laparoscopic colorectal surgery within an enhanced recovery after surgery (ERAS) program. Surg Endosc 2018;32:4003-10. [Crossref] [PubMed]
- Silvestre J, Rebanda J, Lourenço C, et al. Diagnostic accuracy of C-reactive protein and procalcitonin in the early detection of infection after elective colorectal surgery - a pilot study. BMC Infect Dis 2014;14:444. [Crossref] [PubMed]
- Lagoutte N, Facy O, Ravoire A, et al. C-reactive protein and procalcitonin for the early detection of anastomotic leakage after elective colorectal surgery: pilot study in 100 patients. J Visc Surg 2012;149:e345-9. [Crossref] [PubMed]
- Oberhofer D, Rumenjak V, Lazić J, et al. Inflammatory indicators in patients after surgery of the large intestine. Acta Med Croatica 2006;60:429-33. [PubMed]
- Garcia-Granero A, Frasson M, Flor-Lorente B, et al. Procalcitonin and C-reactive protein as early predictors of anastomotic leak in colorectal surgery: a prospective observational study. Dis Colon Rectum 2013;56:475-83. [Crossref] [PubMed]
- Giaccaglia V, Salvi PF, Antonelli MS, et al. Procalcitonin reveals early dehiscence in colorectal surgery: the PREDICS study. Ann Surg 2016;263:967-72. [Crossref] [PubMed]
- Aoki Y, Taniai N, Yoshioka M, et al. Serum procalcitonin concentration within 2 days postoperatively accurately predicts outcome after liver resection. Clin Chem Lab Med 2018;56:1362-72. [Crossref] [PubMed]
- Clementi A, Virzì GM, Muciño-Bermejo MJ, et al. Presepsin and procalcitonin levels as markers of adverse postoperative complications and mortality in cardiac surgery patients. Blood Purif 2019;47:140-8. [Crossref] [PubMed]
- Sint A, Lutz R, Assenmacher M, et al. Monocytic HLA-DR expression for prediction of anastomotic leak after colorectal surgery. J Am Coll Surg 2019;229:200-9. [Crossref] [PubMed]
- Prkno A, Wacker C, Brunkhorst FM, et al. Procalcitonin-guided therapy in intensive care unit patients with severe sepsis and septic shock--a systematic review and meta-analysis. Crit Care 2013;17:R291. [Crossref] [PubMed]
- Mahmutaj D, Krasniqi S, Braha B, et al. The predictive role of procalcitonin on the treatment of intra-abdominal infections. Open Access Maced J Med Sci 2017;5:909-14. [Crossref] [PubMed]
- Huang TS, Huang SS, Shyu YC, et al. A procalcitonin-based algorithm to guide antibiotic therapy in secondary peritonitis following emergency surgery: a prospective study with propensity score matching analysis. PLoS One 2014;9:e90539. [Crossref] [PubMed]
- Hochreiter M, Köhler T, Schweiger AM, et al. Procalcitonin to guide duration of antibiotic therapy in intensive care patients: a randomized prospective controlled trial. Crit Care 2009;13:R83. [Crossref] [PubMed]
- Schroeder S, Hochreiter M, Koehler T, et al. Procalcitonin (PCT)-guided algorithm reduces length of antibiotic treatment in surgical intensive care patients with severe sepsis: results of a prospective randomized study. Langenbecks Arch Surg 2009;394:221-6. [Crossref] [PubMed]
- Shehabi Y, Sterba M, Garrett PM, et al. Procalcitonin algorithm in critically ill adults with undifferentiated infection or suspected sepsis. A randomized controlled trial. Am J Respir Crit Care Med 2014;190:1102-10. [Crossref] [PubMed]
- Rhodes A, Evans LE, Alhazzani W, et al. Surviving sepsis campaign: international guidelines for management of sepsis and septic shock: 2016. Crit Care Med 2017;45:486-552. [Crossref] [PubMed]
- Kalil AC, Metersky ML, Klompas M, et al. Management of adults with hospital-acquired and ventilator-associated pneumonia: 2016 clinical practice guidelines by the Infectious Diseases Society of America and the american thoracic society. Clin Infect Dis 2016;63:e61-111. [Crossref] [PubMed]
- Meisner M, Lohs T, Huettemann E, et al. The plasma elimination rate and urinary secretion of procalcitonin in patients with normal and impaired renal function. Eur J Anaesthesiol 2001;18:79-87. [Crossref] [PubMed]
- Grace E, Turner RM. Use of procalcitonin in patients with various degrees of chronic kidney disease including renal replacement therapy. Clin Infect Dis 2014;59:1761-7. [Crossref] [PubMed]
- Herget-Rosenthal S, Klein T, Marggraf G, et al. Modulation and source of procalcitonin in reduced renal function and renal replacement therapy. Scand J Immunol 2005;61:180-6. [Crossref] [PubMed]
- Dahaba AA, Rehak PH, List WF. Procalcitonin and C-reactive protein plasma concentrations in nonseptic uremic patients undergoing hemodialysis. Intensive Care Med 2003;29:579-83. [Crossref] [PubMed]
- Wu CW, Wu JY, Chen CK, et al. Does procalcitonin, C-reactive protein, or interleukin-6 test have a role in the diagnosis of severe infection in patients with febrile neutropenia? A systematic review and meta-analysis. Support Care Cancer 2015;23:2863-72. [Crossref] [PubMed]
- Sridharan P, Chamberlain RS. The efficacy of procalcitonin as a biomarker in the management of sepsis: slaying dragons or tilting at windmills? Surg Infect (Larchmt) 2013;14:489-511. [Crossref] [PubMed]
- Cui N, Zhang H, Chen Z, et al. Prognostic significance of PCT and CRP evaluation for adult ICU patients with sepsis and septic shock: retrospective analysis of 59 cases. J Int Med Res 2019;47:1573-9. [Crossref] [PubMed]
Cite this article as: Paruk F, Chausse JM. Monitoring the post surgery inflammatory host response. J Emerg Crit Care Med 2019;3:47.