Novel wearable cooling device for early initiation of targeted temperature management in the Emergency Department
Introduction
With an incidence of around 140 per 100,000 people (1), out-of-hospital cardiac arrest represents a major disease burden. In metropolitan cities with developed pre-hospital systems, 9.4–45.5% (2) would go on to achieve return of spontaneous circulation (ROSC). This seemingly optimistic statistic is tempered by the sobering fact that only 0.5–8.5% (2) of patients who achieve ROSC would eventually be discharged alive from the hospital, with good neurological function in less than 3%.
Following two landmark studies (3,4), targeted temperature management (TTM), also previously known as therapeutic hypothermia, forms a critical component of post-resuscitation care. The importance of TTM is reflected by its role in the post-resuscitation care bundles of resuscitation guidelines from the American Heart Association (5), and International Liaison Committee for Resuscitation (6). A standard TTM protocol consists of 3 phases—the induction phase, where the patient is cooled to the target temperature, the maintenance phase, where the patient is kept at the target temperature, and the rewarming phase, where the patient is gradually warmed to normothermia.
The mechanism of TTM and its effect on the post-cardiac arrest syndrome (7) and ischaemic tissue are multifactorial and complex (8), with controversy surrounding the ideal target temperature (9-11) and cooling method (12,13). Although no randomized controlled trial has demonstrated a strong correlation between a shorter time from ROSC to the initiation of TTM or a shorter induction phase to better clinical outcomes, animal studies (14,15) suggest that this is likely. Observational studies (16-18) also support the finding of better outcomes with either a shorter delay from ROSC to initiating TTM or a faster rate of cooling. An inter-trial meta-analysis (19) across 13 different randomized controlled trials concluded that an overall shorter time from ROSC to target temperature results in better outcomes.
Our study aims to evaluate the cooling efficacy of a protocol including a novel surface cooling device in the Emergency Department. We hypothesize that compared to the previous protocol, inclusion of the cooling device would lead to a shorter time from ROSC to the target temperature, and therefore better mortality and neurological outcomes.
Methods
The study was designed as a prospective, single-center pre-post cohort study of cardiac arrest patients with ROSC, for whom TTM was initiated at the Emergency Department (ED) in a tertiary hospital in Singapore.
Prehospital Emergency Care in Singapore is primarily provided by a centralized, government organization (20). Paramedics adopt a “scoop and run” approach towards emergencies and do not pronounce patients dead (except for circumstances like rigor mortis). Therefore, nearly all patients with an out-of-hospital cardiac arrest will be brought to an ED.
Singapore General Hospital is the largest tertiary hospital in the country. It is a Level 1 trauma center, and the ED serves over 125,000 patients annually.
This study was nested within a larger study evaluating the association between post-resuscitation factors and patient outcomes. The period of recruitment was from April 2010 and December 2017, with the intervention introduced in July 2015.
Study participants were post-cardiac arrest patients who had TTM initiated and were subsequently admitted. The inclusion criteria for TTM were patients who (I) had a ROSC time of ≥30 minutes, (II) were aged ≥18 years, (III) remained comatose, or unresponsive with a GCS ≤8, (IV) were hemodynamically stable with systolic blood pressure ≥90 with or without inotropic support, and (V) had arrest of all initial rhythms. Exclusion criteria for TTM were patients who (I) had traumatic causes of arrest, (II) had persistent hypotension despite fluids and/or inotropic support, (III) were not for further intervention, (IV) regained consciousness, (V) were female, aged ≤50 years, and had a positive pregnant test, and/or (VI) were deemed unsuitable candidates by the attending physician. Other exclusion criteria specific to the study were patients who (I) died before admission, (II) had indeterminate cooling methods utilized, (III) had TTM initiated elsewhere and not in the ED, and/or (IV) had TTM prematurely discontinued.
The intervention was a surface cooling device (CarbonCool®, Global Healthcare Pte Ltd), consisting of an external suit (Full Body SuitTM) and cooling pads made of graphite-water material (MPadsTM). The pre-cooled cooling pads are placed into the suit which is worn directly on the patient’s chest, abdomen and thighs (refer to Figure S1). The device does not require an external energy source and is portable, allowing for quick application without much setup. Interventions like further resuscitation, radiological investigations and coronary angiography can take place when the device is on the patient.
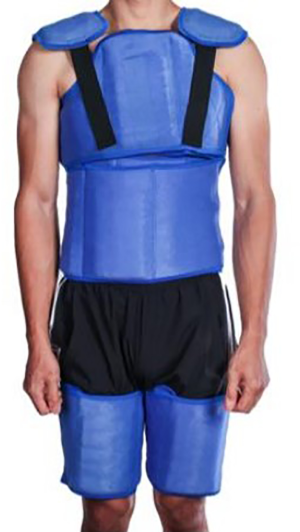
TTM protocol for the pre-intervention, or control period utilized ice packs and cold 0.9% normal saline, with the attending physician determining the appropriate amount. The target temperature was set at 34.0 °C and was followed by further cooling in the intensive care units using standard powered cooling devices. The post-intervention, or intervention period utilized an identical protocol except for the additional use of the surface cooling device. TTM protocols were executed by physicians and nurses trained in the use of the cooling devices and methods. There was no other significant alteration to the post-resuscitation care protocol during the duration of the study period.
The study protocol was approved by SingHealth Centralised Institutional Review Board with waiver of informed consent.
Variables collected for the study followed the Utstein recommendations (21) for reporting and documenting cardiac arrest and resuscitation. The predefined primary outcome assessed was a comparison between the control and intervention periods’ time from ROSC to target temperature (ROSC to TT). This was defined as the duration between the time a patient achieves ROSC to the time the patient’s core temperature reaches the target temperature of 34.0 °C. ROSC was taken as the clinical palpation of a patient’s pulse in the context of resuscitation, and the patient’s core temperature was measured by bladder (Foley catheter temperature sensor, Smiths Medical) or esophageal (Smiths Medical) temperature probes. An interrupted time series graph of the ROSC to TT over the entire study period was used to mitigate the effect of possible time-varying confounders from the intervention.
Clinical secondary outcomes were the two periods’ survival to discharge and neurological outcomes. Survival to discharge referred to a patient surviving the primary event and was ultimately discharged from the hospital alive, regardless of neurological status. The Glasgow-Pittsburg cerebral and overall performance categories (22), determined retrospectively from a careful review of the patient’s medical records, were used to evaluate the neurological status of patients at point of discharge. A score of ≤2 for both categories denoted a good neurological outcome.
Another secondary objective was to evaluate the difference in cooling efficacy between the control and intervention periods, defined as the time from TTM to target temperature (TTM to TT). This was the duration between the initiation of TTM, taken as the logging of the TTM protocol by a clinician, to the target temperature as defined above. As a marker of physician’s barriers to utilizing TTM, the time from ROSC to the initiation of TTM was also measured. Other objectives included recording serious adverse events and incidents of overcooling, defined as a core temperature of <33.0 °C.
A centralised and secured data management platform known as Research Electronic Data Capture (REDCap) System was used to collect the data, which was subsequently analyzed using SPSS version 23.0 (IBM, Chicago IL). Continuous variables are given as medians and interquartile ranges, and categorical variables as frequencies and percentages. For comparisons between the different periods, Pearson’s chi-square for multinomial variables, Fisher’s exact test for binomial variables, and Mann-Whitney U-test for nonparametric continuous variables were used. The statistical significance was set at a two-sided P value of <0.05.
Cooling data logged by temperature probes was collected, then aggregated to produce cooling curves reflecting the mean core temperature over time of patients since starting TTM. The initial cooling rate was determined by finding the formula of the initial best fit curve, then differentiating it for the initial gradient.
Missing values were assumed to be missing at random and handled via pairwise deletion. Post-hoc statistical analysis of missing primary outcome values suggested that it is missing completely at random.
Results
From April 2010 to December 2017, a total of 1,466 patients presented to the Emergency Department of the study centre. Out of 1,466 patients, only 162 survived to admission and met the inclusion criteria for the initiation of TTM. 38 of these patients fell into the exclusion criteria for this study as shown in Figure 1. The remaining 124 patients were enrolled into the study, with 84 enrolled in the control period from April 2010 to July 2015, and 40 enrolled in the intervention period from July 2015 to Dec 2017.
Baseline characteristics and peri-arrest data of the study participants are given in Table 1. There were a few differences between the two periods, with a greater proportion of participants in the intervention period having had a cardiac arrest within the hospital grounds at 17.5% versus control 1.2% (P<0.001) and pre-existing respiratory conditions at 35.0% versus control 17.9% (P=0.043). The intervention period also had higher rates of shockable initial presenting rhythms (ventricular fibrillation, pulseless ventricular tachycardia, and shocks delivered via automated external defibrillation) at 37.5% versus control 25.0% (P=0.05), although this was complicated by a high proportion of unknown initial rhythms in the control period (15.5%). Rates of witnessed collapse, bystander cardiopulmonary resuscitation, initial rhythms, prehospital defibrillation, a final diagnosis of a cardiac cause of arrest and the median time to ROSC were not significantly different between the two periods.
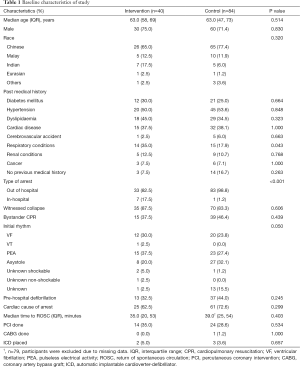
Full table
Study outcomes are shown in Table 2. Study participants in the intervention period had a significantly decreased median time from ROSC to TT at 119 [interquartile range (IQR), 65–250] minutes versus control 482 (IQR, 356–596) minutes (P<0.001). Cooling rates were faster in the intervention period, with a median time of 73 (IQR, 40–150) minutes versus control 142 (IQR, 75–262) minutes (P=0.014) for the initiation of TTM to TT. Median time from ROSC to the initiation of TTM were also markedly different, with the intervention period at 30 (IQR, 6–49) minutes versus control 292 (IQR, 191–384) minutes, (P<0.001).
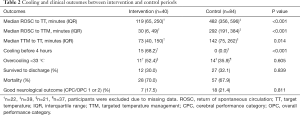
Full table
Secondary outcomes of both periods were similar, with a 30.0% survival to discharge in the intervention period versus control 32.1% (P=0.839) and Glasgow-Pittsburg cerebral and overall performance categories scores of 1 or 2 of 17.5% versus control 21.4% (P=0.811).
There were no reported serious adverse events associated with the device. Rates of overcooling were not significantly different between both periods, at 52.4% in the intervention period versus 35.9% (P=0.605) in the control period.
Aggregate cooling curves for the intervention and control periods are shown in Figure 2. The cooling rates were 2.1 °C/hour for the intervention period and 0.5 °C/hour for the control period. An interrupted time series graph displaying the change in mean time from ROSC to TT on a yearly basis for the duration of the study is shown in Figure 3.
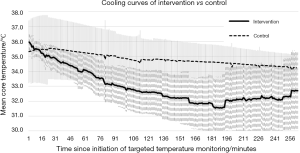
Discussion
The time from ROSC to TT achieved in the intervention period of our study at 119 minutes was lower than or comparable to that of various TTM studies, which ranged from 204 to 480 minutes (3,4,9,12,18). Notably, the timing achieved by our study was under the 180- to 240-minute timeframe suggested by animal studies (14,15) and the 210-minute limit suggested by a meta-analysis (19) to result in better clinical outcomes compared to a more prolonged or delayed cooling time.
Tissue injury occurs after ROSC because of the dual insult of initial ischaemia followed by subsequent reperfusion (23), a set of physiological processes and events which occur when blood flow is restored to ischaemic tissue.
TTM reduces the reperfusion injury that occurs after ROSC, resulting in persistent neuroprotection (14). This is mediated by via various physiological changes (8) such as reducing blood-brain barrier opening, attenuating neutrophil infiltration and thus inflammation, reducing cerebral metabolism, and increasing arterial neuroprotection D1 (24), a lipid modulator which inhibits oxidative stress–induced inflammation and promotes cell survival (25).
Analysing the time from ROSC to TT in terms of its component parts, namely that of the time from ROSC to TTM and the time from TTM to TT, the intervention period faired significantly better for both metrics. However, the relationship between time from ROSC to TT and clinical outcomes is a complex one. A decreased time from ROSC to TTM, or even peri-arrest induction of hypothermia, has been linked to better outcomes in animal (14,15) and in observational studies (16,17). However, the results of randomised controlled trials evaluating the effect of pre-hospital or intra-arrest TTM were less homogenous (26). The use of excessive amounts of intravenous fluids in the process of carrying out rapid cooling results in lower coronary perfusion pressures (27), a potentially harmful effect. By using an external surface cooling device in our study, this deleterious effect is reduced.
It has also been proposed (28) that a longer TTM to TT time is a prognostic factor for better survival and neurological outcomes as it reflects an intact cerebral thermoregulatory function (29). This phenomenon could have accounted for the similar mortality or neurological outcome in our study despite a significant decrease in the time from ROSC to TT, suggesting that the intervention group had patients of poorer prognosis, reflecting a confounding variable in the study. However, the baseline pre- and peri-arrest characteristics which are known prognostic indicators (30) were similar in both periods, making it unlikely that the difference in prognosis was significant enough to cause the difference in TTM to TT observed. The median ROSC to TTM, a metric not influenced by the patient’s prognosis, was also significantly lowered in the intervention period, reflecting an impact of the intervention protocol. Thus, the difference in ROSC to TT time cannot be accounted for solely by the intervention period containing patients with poorer prognosis but must be attributed to the intervention.
Limitations
There were several limitations to our study. The small sample size and presence of missing values led to the study being underpowered to find differences in clinical outcomes despite a large improvement in cooling outcomes. Further studies are needed to show outcome benefit.
A methodological limitation was the use of a pre-post cohort study design, which has the risk of erroneously attributing the difference in cooling outcomes to the effects of the intervention when it could be due to some other unknown variable. A randomised controlled trial would have been ideal for maximizing internal validity.
Another limitation was the time difference between the initiation of TTM and placement of temperature probes in some study participants, perhaps due to the hectic conditions during the post-resuscitation period. This led to instances of overcooling and a lack of initial cooling data for a subset of study participants. Greater staff familiarity with equipment and protocols would reduce its incidence.
Conclusions
Although promising, more research is needed to determine the optimal TTM protocol. The addition of a surface cooling device to an ED’s TTM protocol was associated with a significantly improved time from ROSC to TT and cooling rate compared to the control protocol. As it is reusable and does not require a power source, it has potential to be an affordable solution for pre-hospital and transport cooling.
Acknowledgments
We would like to thank and acknowledge the contributions from all the physicians and nurses from the following departments: Department of Emergency Medicine, Singapore General Hospital; Intensive Care Unit, Department of Respiratory and Critical Care Medicine, Singapore General Hospital; Coronary Care Unit, Department of Cardiology, National Heart Centre Singapore. This study was sponsored by Global Healthcare Pte Ltd. The study sponsor had no involvement in the study design, data collection, data analysis and interpretation, and writing of the manuscript.
Footnote
Conflicts of Interest: MEHO has licensing agreement and patent filing (Application no: 13/047,348) with ZOLL Medical Corporation for a study titled ‘Method of predicting acute cardiopulmonary events and survivability of a patient’. He is also scientific advisor to Global Healthcare, a start-up which develops the Carboncool® suit. The other authors have no conflicts of interest to declare.
Ethical Statement: The authors are accountable for all aspects of the work in ensuring that questions related to the accuracy or integrity of any part of the work are appropriately investigated and resolved. Ethical approval for this study was obtained from Singhealth Centralised Institutional Review Boards (CIRB ref no: 2016/2209) with waiver of informed consent.
References
- Benjamin EJ, Virani SS, Callaway CW, et al. Heart disease and stroke statistics - 2018 update: A report from the American Heart Association. Circulation 2018;137:e67-e492. [Crossref] [PubMed]
- Ong MEH, Shin S, Do , De Souza NNA, et al. Outcomes for out-of-hospital cardiac arrests across 7 countries in Asia: The Pan Asian Resuscitation Outcomes Study (PAROS). Resuscitation 2015;96:100-8. [Crossref] [PubMed]
- Bernard SA, Gray TW, Buist MD, et al. Treatment of Comatose Survivors of out-of-Hospital Cardiac Arrest with Induced Hypothermia. N Engl J Med 2002;346:557-63. [Crossref] [PubMed]
- HACA Study Group. Mild therapeutic hypothermia to improve the neurologic outcome after cardiac arrest. N Engl J Med 2002;346:549-56. [Crossref] [PubMed]
- Callaway CW, Donnino MW, Fink EL, et al. Part 8: Post-cardiac arrest care: 2015 American Heart Association guidelines update for cardiopulmonary resuscitation and emergency cardiovascular care. Circulation 2015;132:S465-82. [Crossref] [PubMed]
- Callaway CW, Soar J, Aibiki M, et al. 2015 International Consensus on Cardiopulmonary Resuscitation and Emergency Cardiovascular Care Science With Treatment Recommendations Part 4: Advanced Life Support. Circulation 2015;132:S84-145. [Crossref] [PubMed]
- Polderman KH, Varon J. Cool hemodynamics - The intricate interplay between therapeutic hypothermia and the post-cardiac arrest syndrome. Resuscitation 2014;85:975-6. [Crossref] [PubMed]
- Gibson A, Andrews PJD. Therapeutic hypothermia, still “too cool to be true?” F1000Prime Rep 2013;5:26. [Crossref] [PubMed]
- Nielsen N, Wetterslev J, Cronberg T, et al. Targeted Temperature Management at 33°C versus 36°C after Cardiac Arrest. N Engl J Med 2013;369:2197-206. [Crossref] [PubMed]
- Fukuda T. Targeted temperature management for adult out-of-hospital cardiac arrest: Current concepts and clinical applications. J Intensive Care 2016;4:30. [Crossref] [PubMed]
- Polderman KH, Varon J. Interpreting the Results of the Targeted Temperature Management Trial in Cardiac Arrest. Ther Hypothermia Temp Manag 2015;5:73-6. [Crossref] [PubMed]
- Glover GW, Thomas RM, Vamvakas G, et al. Intravascular versus surface cooling for targeted temperature management after out-of-hospital cardiac arrest - an analysis of the TTM trial data. Crit Care 2016;20:381. [Crossref] [PubMed]
- Kim KH, Shin S, Do , Song KJ, et al. Cooling methods of targeted temperature management and neurological recovery after out-of-hospital cardiac arrest: A nationwide multicenter multi-level analysis. Resuscitation 2018;125:56-65. [Crossref] [PubMed]
- Colbourne F, Corbett D. Delayed postischemic hypothermia: a six month survival study using behavioral and histological assessments of neuroprotection. J Neurosci 1995;15:7250-60. [Crossref] [PubMed]
- Garnier Y, Pfeiffer D, Jensen A, et al. Effects of mild hypothermia on metabolic disturbances in fetal hippocampal slices after oxygen/glucose deprivation depend on depth and time delay of cooling. J Soc Gynecol Investig 2001;8:198-205. [Crossref] [PubMed]
- Sendelbach S, Hearst MO, Johnson PJ, et al. Effects of variation in temperature management on cerebral performance category scores in patients who received therapeutic hypothermia post cardiac arrest. Resuscitation 2012;83:829-34. [Crossref] [PubMed]
- Lee BK, Jeung KW, Jung YH, et al. Relationship between timing of cooling and outcomes in adult comatose cardiac arrest patients treated with targeted temperature management. Resuscitation 2017;113:135-41. [Crossref] [PubMed]
- Mooney MR, Unger BT, Boland LL, et al. Therapeutic hypothermia after out-of-hospital cardiac arrest: Evaluation of a regional system to increase access to cooling. Circulation 2011;124:206-14. [Crossref] [PubMed]
- Schock RB, Janata A, Peacock WF, et al. Time to Cooling Is Associated with Resuscitation Outcomes. Ther Hypothermia Temp Manag 2016;6:208-17. [Crossref] [PubMed]
- Ng YY. Optimal use of emergency services. Singapore Fam Physcian 2014;40:8-13.
- Perkins GD, Jacobs IG, Nadkarni VM, et al. Cardiac Arrest and Cardiopulmonary Resuscitation Outcome Reports: Update of the Utstein Resuscitation Registry Templates for Out-of-Hospital Cardiac Arrest. Resuscitation 2015;96:328-40. [Crossref] [PubMed]
- Edgren E, Hedstrand U, Kelsey S, et al. Assessment of neurological prognosis in comatose survivors of cardiac arrest. Lancet 1994;343:1055-9. [Crossref] [PubMed]
- Pundik S, Xu K, Sundararajan S. Reperfusion brain injury: Focus on cellular bioenergetics. Neurology 2012;79:S44-51. [Crossref] [PubMed]
- White CJ, Marcheselli VL, Janata A, et al. Lipid Mediators as Novel Biomarkers and Surrogate Indicators of Neurologic Recovery after Cardiac Arrest in a Hypothermic Swine Model. Circulation 2006;114:1203.
- Belayev L, Freitas RS, Marcell SJ, et al. In: Caplan LR, Biller J, Leary MC, et al. editors. Primer on Cerebrovascular Diseases. 2nd ed. San Diego: Academic Press, 2017;256-60.
- Scolletta S, Taccone FS, Nordberg P, et al. Intra-arrest hypothermia during cardiac arrest: A systematic review. Crit Care 2012;16:R41. [Crossref] [PubMed]
- Yannopoulos D, Zviman M, Castro V, et al. Intra-cardiopulmonary resuscitation hypothermia with and without volume loading in an ischemic model of cardiac arrest. Circulation 2009;120:1426-35. [Crossref] [PubMed]
- Perman SM, Ellenberg JH, Grossestreuer AV, et al. Shorter time to target temperature is associated with poor neurologic outcome in post-arrest patients treated with targeted temperature management. Resuscitation 2015;88:114-9. [Crossref] [PubMed]
- Morrison SF, Nakamura K. Central neural pathways for thermoregulation. Front Biosci (Landmark Ed) 2011;16:74-104. [Crossref] [PubMed]
- Sasson C, Rogers MAM, Dahl J, et al. Predictors of survival from out-of-hospital cardiac arrest a systematic review and meta-analysis. Circ Cardiovasc Qual Outcomes 2010;3:63-81. [Crossref] [PubMed]
Cite this article as: Yap LG, Shahidah N, Pothiawala S, Tan KBK, Wong ASL, Sewa DW, Lim ETS, Chin CT, Huang W, Ong MEH. Novel wearable cooling device for early initiation of targeted temperature management in the Emergency Department. J Emerg Crit Care Med 2020;4:13.