Effect of intracranial pressure monitoring on mortality following severe traumatic brain injury in Thailand: propensity score matching methods
Highlight box
Key findings
• There was no statistically significant reduction in mortality observed in severe traumatic brain injury (TBI) patients when intracranial pressure (ICP) monitoring was implemented.
What is known and what is new?
• There has been a discussion on the impact of ICP monitoring on survival in the literature review and ICP monitoring catheters obtained treatment costs, which may become an economic burden in certain regions, especially in low- and middle-income countries. Patients with severe traumatic brain injuries did not significantly benefit from ICP monitoring in terms of survival using various propensity score matching analysis.
What is the implication, and what should change now?
• The survival benefits of implementing ICP monitoring should be investigated more in the future, considering both efficacy and health economics. In a limited resources setting, the imaging-clinical examination protocol may be a viable alternative for improving functional outcomes in patients with severe TBI.
Introduction
Traumatic brain injury (TBI) is one of the most serious public health issues (1-3). In 2021, the Centers for Disease Control and Prevention estimated that 69,473 Americans died as a result of TBI (1). In addition, 35% of TBI-related deaths in the United States were caused by suicide, whereas road traffic injury was the leading cause of TBI-related mortality in Thailand (2,3). Tanaboriboon et al. found an increase in TBI-related mortality, rising from 3.9–16.0 per 100,000 population between 1983–1992 to 16.3–28.2 per 100,000 population between 1997–2002 (4). In a prior study in Thailand, mild, moderate, and severe cases of pediatric TBI were found according to 84.4%, 7.5%, and 8.1%, respectively (5), while mild, moderate, and severe TBIs in patients aged 18 years or older accounted for 92.7%, 3.6%, and 3.6%, respectively (6). Therefore, the severity of TBI has been reported to be a predictor associated with clinical outcomes and quality of life based on prior studies of intracranial hypertension (7,8). Hence, intracranial pressure (ICP) monitoring is a fundamental procedure that has been suggested for understanding intracranial hemodynamics and guiding treatments to enhance neurological outcomes, especially in cases of severe TBI.
From established guidelines, ICP monitoring has been recommended to be performed on intensive care patients with severe TBI because ICP >22 mmHg is related to increasing mortality (9,10). However, the effect of ICP monitoring on morality has been debated in prior studies (11,12). Han et al. conducted a systematic review and meta-analysis of 6 randomized controlled trials and 12 cohort studies, which found that ICP monitoring did not significantly decrease hospital mortality in patients with severe TBI (13). Conversely, Shibahashi et al. used a Japanese countrywide database to study the benefits of ICP monitoring and discovered that it was related to significantly lower in-hospital mortality (14). Furthermore, Shen et al. carried out a systematic review and discovered that ICP monitoring significantly reduced hospital mortality (15). Although ICP monitoring has had an inconsistent effect on outcomes, this procedure is commonly utilized in practice. Consequently, treatment costs increased from ICP monitoring catheters, which can become an economic burden in certain regions, particularly in low- and middle-income countries (LMIC). For TBI patients in Thailand, ICP monitoring is one of the procedures that causes out-of-pocket expenses because the cost of the intraparenchymal ICP catheter has been not covered by Thailand’s public health insurance schemes (16,17). Therefore, this procedure has been considered and weighed against the benefits of intervention as well as the subsequent economic burden.
There remains a lack of strong evidence and randomized-controlled studies regarding the impact of ICP monitoring on mortality (9,18). Propensity score matching (PSM) is one of the methods to control the confounders or preoperative imbalanced covariates in observational studies. Agrawal et al. employed propensity score analysis to determine the effect of ICP monitoring on hospital mortality and functional outcomes in patients with severe TBI (18). They found that ICP monitoring reduced hospital mortality but had no influence on 6-month functional outcomes. Due to the gap in knowledge, the effect of ICP monitoring is challenging to estimate using PSM, and the results could be used to assist neurosurgeons in LMIC in making treatment decisions for TBI patients. Thus, the objectives of the present study were to evaluate the relationship between ICP monitoring and hospital mortality in cases of severe TBI using various PSM methods. Additionally, the secondary objective was to estimate the factors associated with ICP monitoring. We present this article in accordance with the STROBE reporting checklist (available at https://jeccm.amegroups.com/article/view/10.21037/jeccm-23-109/rc).
Methods
Study designs and study population
This study utilized a retrospective cohort approach based on data from medical records at a level 1 trauma center in Southern Thailand. Patients aged 15 years or older who had experienced severe TBI (GCS score ≤8) and had undergone intraparenchymal ICP monitoring (Codman microsensor) between January 2014 and January 2023 were included in the study. Demographic data, neuroimaging findings, treatment, operation, and mortality rates were all collected.
Patients who died before arriving at the emergency department, did not have a preoperative cranial computed tomography (CT) scan, had ventriculostomy (intraventricular monitoring), or whose medical data was unavailable were excluded.
Operational definition
The demographics, cranial CT scan findings, and treatment on admission were reviewed for analysis. Because hypotension produces a misinterpretation of the GCS score due to inadequate cerebral perfusion, the GCS score collected in the current investigation was the patient’s GCS score with stable vital signs after resuscitation at the emergency department. Additionally, road traffic injury was defined as the mechanism of injury in cases involving car crashes, motorcycle crashes and pedestrian injuries (19). Two neurosurgeons assessed the cranial CT findings, type of intracranial injuries, midline displacement, and obliteration of the basal cistern. According to Vieira et al., diffuse axonal injury (DAI) was defined as the presence of DAI symptoms that were consistent with neuroimaging findings (20).
Standard treatment
Although no defined TBI procedure was employed, the following standard TBI management concepts were followed. Patients with severe TBI were admitted to the hospital’s surgical intensive care unit or neuro-trauma intensive care unit. Patients with a GCS score of 8 or less were intubated, and normal saline was used to maintain euvolemia status in patients both with/without ICP monitoring groups. In the ICP monitoring group, the goal ICP was 20–22 mmHg based on 2007 and 2016 Brain Trauma Foundation guidelines (9,21). When patients did not receive ICP monitoring, the treatment of cerebral edema and elevated ICP was based on a reassessment of the patient’s neurological status and serial cranial CT scans. Osmotherapy was used to avoid complications such as renal failure and hypotension in both groups. Temporary hyperventilation to partial pressure of carbon dioxide (PCO2) 30 mmHg was used only as a rescue measure in cases of cerebral herniation or neurological deterioration by elevated ICP. In addition, maintaining normothermia (body temperature <38 ℃) also included taking acetaminophen on a regular basis and using cooling blankets.
Ethical considerations
The study was conducted in accordance with the Declaration of Helsinki (as revised in 2013). The present study was approved by the Human Research Ethics Committee (REC.66-201-10-1). Because this study utilized a retrospective study design, informed consent from patients was not required. However, patient identification numbers were encoded before analysis.
Statistical analysis
According to their procedure, patients were divided into two groups: ICP monitoring and non-ICP monitoring. Clinical features of patients, with and without ICP monitoring, were compared using a Chi-square test, independent t-test, and standardized mean difference (SMD). In detail, SMD greater than 0.1 is a recommended threshold for declaring an imbalance of clinical characteristics between groups (22,23).
Initially, the traditional method of binary logistic regression was performed to analyze the effect of ICP monitoring on mortality using both univariate analysis and multivariable analysis. In univariate analysis, factors with a P value less than 0.1 were designated as candidate factors; these variables were analyzed using multivariable analysis with the backward stepwise procedure. The final model was selected based on the lowest Akaike information criterion (AIC) value. Finally, the parameters of the final model were integrated to generate an ICP monitoring propensity score for each patient (24).
In this study, the authors utilized the PSM method to address the issue of selection bias in observational data collection. To control the bias of treatment assignment and adjust the baseline characteristics between the two groups, the following various PSM methods were utilized: exact matching, nearest neighbor matching with a ratio of 1:1, nearest neighbor matching with a ratio of 2:1, nearest neighbor matching with a propensity score caliper of 0.2, optimal matching, and Mahalanobis metric matching (22,23). After PSM, the effect of ICP monitoring on mortality was estimated by univariate analysis and multivariable analysis with covariates adjustment; crude odds ratio (OR) and adjusted OR were reported in each PSM method.
Because PSM had limitations from reducing the original sample size, the authors also performed a propensity score covariate adjustment to determine the effect of ICP monitoring on mortality in severe TBI. Hence, descriptive analysis was used to analyze both matched and unmatched datasets, as well as baseline clinical features, which were provided as proportions with percentages and mean with standard deviation (SD). In addition, the Kolmogorov-Smirnov test was performed for the normality test of continuous variables. All analyses were carried out with the R version 4.4.0 software (R Foundation, Vienna, Austria), and PSM was carried out with the MatchIt package (25).
Results
Unmatched cohort
Among 849 patients with GCS scores of 8 or less, the baseline characteristics for patients in the unmatched cohorts were presented, as shown in Table 1. For the normal distribution test of various continuous variables, the P value of the Kolmogorov-Smirnov test was more than 0.05 of all preferred normal distribution of data. The mean age of patients was 37.51±19.66 years), and 21.0% of those in the present study were female. Road traffic injuries comprising car crashes, motorcycle crashes and pedestrian injuries were the most common mechanisms. Alcohol was detected in 35.1% (143/407) of blood samples, and the mean alcoholic level was 68.60 mg% (SD 106.36). ICP monitoring was performed on 35 TBI patients, and hospital mortality rate was observed in 31.2% of total cases. In detail, 8 (22.9%) patients in the ICP monitoring group died in the hospital, while fatal cases were found in 31.6% (257/557) of the non-ICP monitoring group.
Table 1
Factor | ICP monitoring | P value* | SMD | ||
---|---|---|---|---|---|
No (n=814) | Yes (n=35) | ||||
Sex, n (%) | 0.57 | 0.10 | |||
Male | 642 (78.9) | 29 (82.9) | |||
Female | 172 (21.1) | 6 (17.1) | |||
Age, years, mean (SD) | 37.81 (19.66) | 30.43 (18.47) | 0.02 | 0.38 | |
Mechanism of injury, n (%) | 0.23 | 0.16 | |||
Fall from height | 117 (14.4) | 3 (8.6) | |||
MCA | 521 (64.0) | 28 (80.0) | |||
Car crash | 69 (8.5) | 1 (2.9) | |||
Pedestrian | 33 (4.1) | 0 | |||
Object struck to head | 4 (0.5) | 0 | |||
Penetrating | 18 (2.2) | 0 | |||
Blast | 20 (2.5) | 0 | |||
Assault | 18 (2.2) | 1 (2.9) | |||
Bicycle | 4 (0.5) | 0 | |||
Other | 10 (1.2) | 2 (5.7) | |||
Multiple trauma, n (%) | 639 (78.5) | 28 (80.0) | 0.36 | 0.14 | |
Aspirin, n (%) | 13 (1.6) | 1 (2.9) | 0.56 | 0.08 | |
Clopidogrel, n (%) | 7 (0.9) | 0 | 0.58 | 0.13 | |
Warfarin, n (%) | 6 (0.7) | 0 | 0.61 | 0.12 | |
Thrombocytopenia, n (%) | 3 (0.4) | 0 | 0.71 | 0.08 | |
Seizure, n (%) | 48 (5.9) | 3 (8.6) | 0.51 | 0.10 | |
Hypotension, n (%) | 130 (16.0) | 1 (2.9) | 0.03 | 0.46 | |
Bradycardia, n (%) | 21 (2.6) | 0 | 0.33 | 0.23 | |
Pupillary light reflex, n (%) | 0.01 | 0.44 | |||
Fixed both eyes | 157 (19.3) | 4 (11.4) | |||
React one eyes | 149 (18.3) | 13 (37.1) | |||
React both eyes | 508 (62.4) | 18 (51.4) | |||
Time from field to hospital arrival, min, mean (SD) | 70.5 (51.2) | 68.6 (50.3) | 0.37 | 0.11 | |
Time from hospital arrival to ICP monitoring, min, mean (SD) | 160.3 (87.3) | 161.2 (85.1) | 0.41 | 0.12 | |
Alcoholic level, mg%, median (IQR) | 58.40 (149.95) | 56.24 (84.80) | 0.49 | 0.12 | |
Injury Severity Score, mean (SD) | 25.67 (11.73) | 27.59 (12.25) | 0.40 | 0.16 | |
Abbreviated Injury Scale of head, mean (SD) | 3.98 (1.30) | 4.37 (0.83) | 0.12 | 0.35 | |
Skull fracture, n (%) | 125 (15.4) | 2 (5.7) | 0.11 | 0.31 | |
Basilar skull fracture, n (%) | 189 (23.2) | 10 (28.6) | 0.46 | 0.12 | |
EDH, n (%) | 137 (16.8) | 8 (22.9) | 0.35 | 0.15 | |
Thickness of EDH, mm, mean (SD) | 10.86 (13.01) | 8.87 (4.45) | 0.19 | 0.61 | |
SDH, n (%) | 456 (56.0) | 20 (57.1) | 0.89 | 0.02 | |
Thickness of SDH, mm, mean (SD) | 7.81 (5.71) | 6.12 (4.99) | 0.18 | 0.31 | |
Contusion/intracerebral hematoma, n (%) | 323 (39.7) | 20 (57.1) | 0.04 | 0.35 | |
Diameter of contusion, mm, mean (SD) | 17.35 (16.71) | 15.46 (15.77) | 0.62 | 0.11 | |
SAH, n (%) | 359 (44.1) | 18 (51.4) | 0.39 | 0.14 | |
IVH, n (%) | 132 (16.2) | 8 (22.9) | 0.30 | 0.16 | |
Brainstem hematoma, n (%) | 19 (2.3) | 2 (5.7) | 0.20 | 0.17 | |
DAI, n (%) | 84 (10.3) | 14 (40.0) | <0.001 | 0.72 | |
Basal cistern, n (%) | 0.04 | 0.38 | |||
Patent | 541 (66.5) | 29 (82.9) | |||
Obliterated | 273 (33.5) | 6 (17.1) | |||
Midline shift, mm, mean (SD) | 3.25 (5.41) | 1.37 (3.53) | 0.005 | 0.41 |
*, P value of Chi-square test and t-test. ICP, intracranial pressure; SMD, standardized mean difference; SD, standard deviation; MCA, motorcycle accident; IQR, interquartile range; EDH, epidural hematoma; SDH, subdural hematoma; SAH, subarachnoid hemorrhage; IVH, intraventricular hemorrhage; DAI, diffuse axonal injury.
The mean age of the non-ICP monitoring group was significantly higher than that of the ICP monitoring group, while the average midline shift on cranial CT scan of non-ICP monitoring was higher than that of other group. The proportions of hypotension, pupillary light reflex, cerebral contusion/intracerebral hematoma, DAI, and obliterated basal cistern were significantly different between the two groups by Chi-square test. Additionally, SMD greater than 0.1 indicates an imbalance of baseline characteristics between the two groups, and the baseline characteristics that had an SMD greater than 0.1 were as follows: age, mechanism of injury, clopidogrel, warfarin, hypotension, bradycardia, pupillary light reflex, alcoholic level, Injury Severity Score (ISS), Abbreviated Injury Scale (AIS) of the head, skull fracture, basilar skull fracture, epidural hematoma (EDH), the thickness of EDH, the thickness of SDH, contusion/intracerebral hematoma, diameter of contusion/intracerebral hematoma, subarachnoid hemorrhage (SAH), intraventricular hemorrhage (IVH), brainstem hematoma, DAI, basal cistern obliteration, and midline shift.
Factors associated with the ICP monitoring procedure
Preoperative characteristics were analyzed to explore which factors were significantly related to the ICP monitoring procedure. Using univariate analysis, the candidate variables were age, hypotension, pupillary light reflex, contusion/intracerebral hematoma, DAI, basal cistern obliteration, and midline shift on cranial CT scan. As shown in Table 2, age, hypotension, pupillary light reflex, midline shift, and DAI were included in the final model that had the lowest AIC value by multivariable analysis with a backward stepwise approach. Finally, these factors were the potential confounders that were combined to generate a propensity score of ICP monitoring for each patient.
Table 2
Factor | Univariate analysis | Multivariable analysis | |||
---|---|---|---|---|---|
Odds ratio (95% CI) | P value | Odds ratio (95% CI) | P value | ||
Sex | |||||
Male | Ref | ||||
Female | 0.77 (0.29–1.77) | 0.57 | |||
Age, years | 0.98 (0.96–1.00) | 0.03 | 0.98 (0.96–1.00) | 0.06 | |
Road traffic injury* | 1.48 (0.61–3.62) | 0.38 | |||
GCS score at emergency department | 0.97 (0.81–1.16) | 0.73 | |||
Aspirin* | 1.81 (0.23–14.26) | 0.57 | |||
Seizure* | 1.50 (0.44–5.06) | 0.51 | |||
Hypotension* | 0.15 (0.02–1.14) | 0.06 | 0.16 (0.01–0.82) | 0.08 | |
Pupillary light reflex | |||||
Fixed both eyes | Ref | Ref | |||
React one eyes | 3.42 (1.09–10.74) | 0.03 | 2.19 (0.71–8.29) | 0.20 | |
React both eyes | 1.39 (0.46–4.17) | 0.55 | 0.77 (0.26–2.85) | 0.66 | |
Alcoholic level, mg% | 1.00 (1.00–1.03) | 0.48 | |||
Injury Severity Score | 1.01 (0.98–1.05) | 0.40 | |||
Abbreviated Injury Scale of head | 1.25 (0.94–1.67) | 0.12 | |||
Skull fracture* | 0.33 (0.08–1.41) | 0.13 | |||
Basilar skull fracture* | 1.32 (0.62–2.80) | 0.46 | |||
EDH* | 1.46 (0.65–3.29) | 0.35 | |||
SDH* | 1.05 (0.53–2.07) | 0.89 | |||
Contusion/intracerebral hematoma | 2.03 (1.02–4.02) | 0.04 | |||
SAH* | 1.34 (0.68–2.64) | 0.39 | |||
IVH* | 1.53 (0.68–3.44) | 0.30 | |||
Brainstem hematoma* | 1.48 (0.64–2.24) | 0.35 | |||
DAI* | 5.79 (2.84–11.82) | <0.001 | 4.11 (1.90–8.66) | <0.001 | |
Basal cistern | |||||
Patent | Ref | ||||
Obliterated | 0.41 (0.41–1.00) | 0.05 | |||
Midline shift-mm | 0.90 (0.81–1.00) | 0.05 | 0.90 (0.79–1.00) | 0.08 |
*, data show only “yes group” while reference groups (no group) are hidden. CI, confidence interval; GCS, Glasgow Coma Scale; EDH, epidural hematoma; SDH, subdural hematoma; SAH, subarachnoid hemorrhage; IVH, intraventricular hemorrhage; DAI, diffuse axonal injury.
Matched cohort
Before analyzing the impact of ICP monitoring on mortality, numerous PSM techniques were employed. As a result, the sample sizes of the matched cohort for each PSM method varied subtly. Table 3 demonstrates the matched datasets for exact matching and nearest neighbor matching with a ratio of 1:1, whereas the matched datasets for other PSM are presented in the Tables S1-S4. Almost all baseline characteristics after PSM were adjusted and SMD less than 0.1, particularly factors associated with the ICP monitoring procedure. The love plots graphically display covariate balance before and after the various matching adjustments, as shown in Figure 1A-1F. In detail, SMDs of various covariates after PSMs ranged from −0.1 to 0.1, which was within the zone of two dashed lines. Moreover, the SMD of covariates was 0 in the exact matching.
Table 3
Factor | Exact matching | NN with ratio 1:1 | |||||||
---|---|---|---|---|---|---|---|---|---|
No (n=31) | Yes (n=15) | P value* | SMD | No (n=35) | Yes (n=35) | P value* | SMD | ||
Sex (female), n (%) | 5 (16.1) | 4 (26.7) | 0.65 | 0.25 | 5 (14.3) | 6 (17.1) | >0.99 | 0.07 | |
Age, years, mean (SD) | 22.52 (15.37) | 22.67 (16.45) | 0.97 | 0.009 | 30.37 (15.86) | 30.43 (18.47) | 0.98 | 0.003 | |
Road traffic injury, n (%) | 25 (80.6) | 14 (93.3) | 0.49 | 0.38 | 30 (85.7) | 29 (82.9) | >0.99 | 0.07 | |
Aspirin, n (%) | 0 | 1 (6.7) | 0.70 | 0.37 | 0 | 1 (2.9) | >0.99 | 0.24 | |
Clopidogrel, n (%) | 0 | 0 | – | <0.001 | 0 | 0 | – | <0.001 | |
Warfarin, n (%) | 0 | 0 | – | <0.001 | 0 | 0 | – | <0.001 | |
Thrombocytopenia, n (%) | 0 | 0 | – | <0.001 | 0 | 0 | – | <0.001 | |
Seizure, n (%) | 3 (9.7) | 1 (6.7) | >0.99 | 0.11 | 6 (17.1) | 3 (8.6) | 0.47 | 0.25 | |
Hypotension, n (%) | 0 | 0 | – | <0.001 | 1 (2.9) | 1 (2.9) | >0.99 | 0.24 | |
Bradycardia, n (%) | 0 | 0 | – | <0.001 | 1 (2.9) | 0 | >0.99 | 0.24 | |
Pupillary light reflex, n (%) | 0.31 | 0.45 | 0.58 | 0.24 | |||||
Fixed both eyes | 1 (3.2) | 1 (6.7) | 5 (14.3) | 4 (11.4) | |||||
React one eyes | 2 (6.5) | 3 (20.0) | 9 (25.7) | 13 (37.1) | |||||
React both eyes | 28 (90.3) | 11 (73.3) | 21 (60.0) | 18 (51.4) | |||||
Alcoholic level, mg%, mean (SD) | 59.69 (85.53) | 58.07 (120.75) | 0.95 | 0.01 | 69.80 (111.29) | 56.25 (108.43) | 0.60 | 0.12 | |
Injury Severity Score, mean (SD) | 19.81 (11.06) | 30.50 (13.79) | 0.02 | 0.85 | 27.54 (9.39) | 27.59 (12.25) | 0.98 | 0.005 | |
Abbreviated Injury Scale of head, mean (SD) | 3.10 (1.26) | 1.00 (0) | 0.007 | 1.032 | 3.88 (1.08) | 4.37 (0.84) | 0.07 | 0.51 | |
Skull fracture, n (%) | 4 (12.9) | 2 (13.3) | >0.99 | 0.01 | 3 (8.6) | 2 (5.7) | >0.99 | 0.11 | |
Basilar skull fracture, n (%) | 5 (16.1) | 6 (40.0) | 0.15 | 0.55 | 6 (17.1) | 10 (28.6) | 0.39 | 0.27 | |
EDH, n (%) | 7 (22.6) | 5 (33.3) | 0.67 | 0.24 | 6 (17.1) | 8 (22.9) | 0.76 | 0.14 | |
Thickness of EDH, mm, mean (SD) | 14.57 (10.34) | 7.80 (5.26) | 0.21 | 0.82 | 14.00 (10.49) | 8.88 (4.45) | 0.23 | 0.63 | |
SDH, n (%) | 7 (22.6) | 9 (60.0) | 0.03 | 0.82 | 20 (57.1) | 20 (57.1) | 0.81 | 0.11 | |
Thickness of SDH, mm, mean (SD) | 4.62 (3.50) | 4.25 (1.94) | 0.94 | 0.03 | 5.81 (3.93) | 6.13 (5.00) | 0.81 | 0.07 | |
Contusion/intracerebral hematoma, n (%) | 8 (25.8) | 10 (66.7) | 0.01 | 0.89 | 18 (51.4) | 20 (57.1) | >0.99 | <0.001 | |
Diameter of contusion, mm, mean (SD) | 5.62 (3.20) | 13.83 (14.77) | 0.14 | 0.76 | 14.78 (16.25) | 15.46 (15.78) | 0.89 | 0.04 | |
SAH, n (%) | 6 (19.4) | 8 (53.3) | 0.04 | 0.75 | 17 (48.6) | 18 (51.4) | >0.99 | 0.05 | |
IVH, n (%) | 3 (9.7) | 6 (40.0) | 0.04 | 0.74 | 9 (25.7) | 8 (22.9) | >0.99 | 0.06 | |
Brainstem hematoma, n (%) | 2 (6.5) | 1 (6.7) | >0.99 | 0.009 | 4 (11.4) | 2 (5.7) | 0.66 | 0.20 | |
DAI, n (%) | 7 (22.6) | 6 (40.0) | 0.37 | 0.38 | 17 (48.6) | 14 (40.0) | 0.63 | 0.17 | |
Obliterated basal cistern, n (%) | 1 (3.2) | 1 (6.7) | >0.99 | 0.15 | 9 (25.7) | 6 (17.1) | 0.56 | 0.21 | |
Mean midline shift, mm, mean (SD) | 0.06 (0.36) | 0.13 (0.52) | 0.60 | 0.15 | 1.43 (3.10) | 1.37 (3.53) | 0.94 | 0.01 |
*, P value of Chi-square test and t-test. NN, nearest neighbor matching; SMD, standardized mean difference; SD, standard deviation; EDH, epidural hematoma; SDH, subdural hematoma; SAH, subarachnoid hemorrhage; IVH, intraventricular hemorrhage; DAI, diffuse axonal injury.
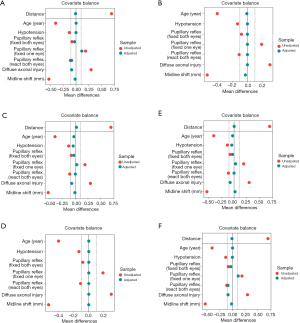
Outcome analysis
Table 4 reveals a comparison of the effect of ICP monitoring on mortality among various PSM methods and traditional approaches. In traditional methods, the crude OR and adjusted OR were 0.64 (95% CI: 0.27–1.37) and 0.88 (95% CI: 0.27–2.63). Additionally, ICP monitoring did not affect mortality for all PSM techniques. However, sample size reduction following PSM may affect the results. Therefore, a propensity score covariate adjustment was conducted to preserve the original dataset. Consequently, ICP monitoring was not associated with mortality using this technique.
Table 4
Approach | Odds ratio (95% CI) | P value |
---|---|---|
Traditional approach | ||
Univariate analysis (crude odds ratio) | 0.64 (0.27–1.37) | 0.30 |
Multivariable analysis with age, GCS at emergency department, hypotension, alcohol level, pupillary light reflex, ISS, head AIS, basilar skull fracture, EDH, contusion, and obliterated basal cistern (adjusted odds ratio) | 0.88 (0.27–2.63) | 0.82 |
Propensity score matching | ||
Exact matching | ||
Crude odds ratio | 0.28 (0.03–1.86) | 0.20 |
Adjusted odds ratio (covariate: GCS at emergency department) | 0.45 (0.05–3.49) | 0.44 |
Nearest neighbor matching with ratio of 1:1 | 0.84 (0.26–2.67) | 0.81 |
Crude odds ratio | 0.84 (0.26–2.67) | 0.77 |
Adjusted odds ratio (covariate: GCS at emergency department) | 0.96 (0.27–3.44) | 0.94 |
Nearest neighbor matching with ratio of 2:1 | ||
Crude odds ratio | 1.17 (0.45–3.03) | 0.74 |
Adjusted odds ratio (covariates: GCS at emergency department, hypotension, head AIS, and basilar skull fracture) | 1.19 (0.35–4.22) | 0.78 |
Nearest neighbor matching with propensity score caliper of 0.2 | ||
Crude odds ratio | 1.00 (0.31–3.26) | 1.00 |
Adjusted odds ratio (covariates: GCS at emergency department, and obliterated basal cistern) | 0.84 (0.20–3.28) | 0.80 |
Optimal matching | ||
Crude odds ratio | 0.84 (0.27–2.65) | 0.77 |
Adjusted odds ratio (covariates: GCS at emergency department, and head AIS) | ||
Mahalanobis metric matching | ||
Crude odds ratio | 1.55 (0.53–4.48) | 0.42 |
Adjusted odds ratio (covariates: GCS at emergency department, hypotension, and midline shift) | 2.54 (0.73–9.94) | 0.15 |
Propensity score covariate adjustment | ||
Crude odds ratio | 1.04 (0.42–2.35) | 0.94 |
Adjusted odds ratio (covariates: age, GCS at emergency department, hypotension, alcohol level, pupillary light reflex, ISS, head AIS, basilar skull fracture, EDH, contusion, and obliterated basal cistern) | 0.87 (0.27–2.63) | 0.81 |
ICP, intracranial pressure; CI, confidence interval; GCS, Glasgow Coma Scale; ISS, Injury Severity Score; AIS, abbreviated Injury Scale; EDH, epidural hematoma.
Discussion
Following the brain trauma foundation guidelines, ICP monitoring has been recommended to be performed on severe TBI patients to reduce in-hospital and 2-week post-injury mortality (9). However, the level of evidence remains limited due to the lack of high-quality evidence reported. From the recommendation, ICP monitoring has been studied and frequently utilized in practice (13,15). However, the number of patients who underwent ICP monitoring procedures was just 4.1% of the present cohort. Because the cost of the ICP monitoring catheter is not covered by all public health insurance schemes in Thailand, ICP monitoring has been conducted less frequently than in previous studies. Moreover, SMD values greater than 0.1 revealed an imbalance in the baseline characteristics of the present cohort.
Accordingly, it was discovered that DAI was the potential bias that prompted neurosurgeons to undertake ICP monitoring. The findings in the present study are in line with a previous study that found that about one-third of individuals with severe TBI who have DAI experienced an increase in ICP (26). This is potentially explained by DAI, which is a severe form of acceleration/deceleration injury with high-speed and high-energy impacts (27,28). Acceleration/deceleration forces successively lead to widespread axonal damage and impaired cerebrovascular reactivity, which cause diffuse brain swelling and gliding contusion with perilesional edema (29). In addition, cerebral edema leads to additional damage to the brain by reducing cortical blood flow and raising ICP (30).
When imbalanced characteristics or preoperative bias are observed in the datasets of observational study, PSM is one of the statistical methods used to address this issue (22,23). Prior studies used propensity score analysis to examine the influence of ICP monitoring on hospital mortality, which found that ICP monitoring reduced hospital mortality (14,18). However, prior systematic review and meta-analysis studies reported a contrary result, showing that ICP monitoring did not have survival benefits in severe TBI (13,15). Yuan et al. analyzed the pooled data from 14 studies and found that ICP monitoring did not significantly reduce the risk of mortality, though the length of hospital stay for the ICP monitoring group was significantly longer compared to the no ICP monitoring group (31). In the present study, the results indicated no significant reduction of hospital mortality in the ICP monitoring group using both traditional methods and various PSM. Following PSM, the sample size was reduced, which had a direct impact on analytical power. To maintain the sample size, propensity score covariate adjustment was applied, and still no significant impact of ICP monitoring on hospital mortality was observed.
In observational data, we observed an even lower rate of ICP monitoring than prior studies that were reported in 30.1–56% of total cases (32-35). This may be explained by the fact that major TBI patients and their relatives could not afford the uncovered cost of the ICP instrument. Therefore, all treated intracranial hypertension protocols were performed without ICP monitoring. Treatment modalities are included in the tiered strategy for the management of increased ICP (36,37). As a result, the majority of the present cohort was treated without ICP monitoring, and there was no significant difference in survival benefit between the two groups.
Imaging-clinical examination (ICE) protocol for severe TBI has been managed without ICP monitoring in LMIC (36-38). Chesnut et al. conducted a multicenter, controlled trial that compared the ICP monitoring-based protocol and the ICP protocol. As a result, there was no significant between-group difference in mortality (36). Moreover, the functional outcome in the use of the ICE protocol group was significantly better than the no protocol group based on a prior study (38). The development of a standard protocol utilizing a variety of noninvasive methods should be carried out in a setting with limited resources in order to enhance the functional outcomes of patients with severe TBI without the need for ICP monitoring.
To the best of the authors’ knowledge, the present study was the first to demonstrate and provide comparative results of ICP monitoring on hospital mortality between various PSM methods. However, it is important to acknowledge the limitations of the present study. Firstly, the present study was conducted using a single-center approach, which may have involved more susceptibility to publication bias (39). A multicenter study enrolls more patients who have undergone ICP monitoring, which will improve the quality and amount of data as well as the dependability of the results (40). Moreover, research using national trauma databases is one alternative option to increase the number of study participants, but the quality of data and in-depth details of individual clinical characteristics and treatment remain challenging to perform (41).
Although PSM methods can resemble a randomized controlled trial, these techniques reduce the sample size after matching the effect to results. As a result, propensity score covariate adjustment was implemented in this study, which did not reduce the sample size and ensured the outcomes remained consistent with PSM methods. In the case of a small sample size, the inverse probability treatment weights method is one of the important techniques for comparing the effectiveness of different treatments without reducing the size of the group (42). Therefore, propensity score weighting should be performed to estimate outcomes in the future. We observed no association between ICP monitoring and mortality in this study. However, future studies that research adjusted treatment methods based on ICP monitoring should investigate the effect of these procedures as multicenter or national database studies.
Finally, the lack of socioeconomic profiles was one of the limitations of the present study. The cost of ICP monitoring catheters can lead to economic burdens. In the future, socioeconomic profiles, as well as direct, indirect, and intangible costs, should be corrected for research focused on the health economic evaluation of this invasive procedure (43).
Conclusions
In summary, the survival benefits of using ICP monitoring should be investigated more in the future, considering both efficacy and health economics aspects. The application of the ICE protocol may be an alternate strategy to enhance functional outcomes for patients with severe TBI in settings with limited resources.
Acknowledgments
Funding: None.
Footnote
Reporting Checklist: The authors have completed the STROBE reporting checklist. Available at https://jeccm.amegroups.com/article/view/10.21037/jeccm-23-109/rc
Data Sharing Statement: Available at https://jeccm.amegroups.com/article/view/10.21037/jeccm-23-109/dss
Peer Review File: Available at https://jeccm.amegroups.com/article/view/10.21037/jeccm-23-109/prf
Conflicts of Interest: Both authors have completed the ICMJE uniform disclosure form (available at https://jeccm.amegroups.com/article/view/10.21037/jeccm-23-109/coif). The authors have no conflicts of interest to declare.
Ethical Statement: The authors are accountable for all aspects of the work in ensuring that questions related to the accuracy or integrity of any part of the work are appropriately investigated and resolved. The study was conducted in accordance with the Declaration of Helsinki (as revised in 2013). The present study was approved by the Human Research Ethics Committee (REC.66-201-10-1). Because this study utilized a retrospective study design, informed consent from patients was not required. However, patient identification numbers were encoded before analysis.
Open Access Statement: This is an Open Access article distributed in accordance with the Creative Commons Attribution-NonCommercial-NoDerivs 4.0 International License (CC BY-NC-ND 4.0), which permits the non-commercial replication and distribution of the article with the strict proviso that no changes or edits are made and the original work is properly cited (including links to both the formal publication through the relevant DOI and the license). See: https://creativecommons.org/licenses/by-nc-nd/4.0/.
References
- Centers for Disease Control and Prevention. Traumatic Brain Injury & Concussion. 2023 [cited 2023 Jul 5]. Available online: https://www.cdc.gov/traumaticbraininjury/data/
- Centers for Disease Control and Prevention. TBI surveillance report 2018-2019. 2023 [cited 2023 Jul 5]. Available online: https://www.cdc.gov/traumaticbraininjury/pdf/TBI-surveillance-report-2018-2019-508.pdf
- Phuenpathom N, Tiensuwan M, Ratanalert S, et al. The changing pattern of head injury in Thailand. J Clin Neurosci 2000;7:223-5. [Crossref] [PubMed]
- Tanaboriboon Y, Satiennam T. Traffic Accidents in Thailand. IATSS Research 2005;29:88-100. [Crossref]
- Tunthanathip T, Phuenpathom N. Impact of Road Traffic Injury to Pediatric Traumatic Brain Injury in Southern Thailand. J Neurosci Rural Pract 2017;8:601-8. [Crossref] [PubMed]
- Tunthanathip T, Oearsakul T, Tanvejsilp P, et al. Predicting the Health-related Quality of Life in Patients Following Traumatic Brain Injury. Surg J (N Y) 2021;7:e100-10. [Crossref] [PubMed]
- Andrews PJ, Sleeman DH, Statham PF, et al. Predicting recovery in patients suffering from traumatic brain injury by using admission variables and physiological data: a comparison between decision tree analysis and logistic regression. J Neurosurg 2002;97:326-36. [Crossref] [PubMed]
- Badri S, Chen J, Barber J, et al. Mortality and long-term functional outcome associated with intracranial pressure after traumatic brain injury. Intensive Care Med 2012;38:1800-9. [Crossref] [PubMed]
- Carney N, Totten AM, O'Reilly C, et al. Guidelines for the Management of Severe Traumatic Brain Injury, Fourth Edition. Neurosurgery 2017;80:6-15.
- Balestreri M, Czosnyka M, Hutchinson P, et al. Impact of intracranial pressure and cerebral perfusion pressure on severe disability and mortality after head injury. Neurocrit Care 2006;4:8-13. [Crossref] [PubMed]
- Stocchetti N, Poole D, Okonkwo DO. Intracranial pressure thresholds in severe traumatic brain injury: we are not sure: Prudent clinical practice despite dogma or nihilism. Intensive Care Med 2018;44:1321-3. [Crossref] [PubMed]
- Woods KS, Horvat CM, Kantawala S, et al. Intracranial and Cerebral Perfusion Pressure Thresholds Associated With Inhospital Mortality Across Pediatric Neurocritical Care. Pediatr Crit Care Med 2021;22:135-46. [Crossref] [PubMed]
- Han J, Yang S, Zhang C, et al. Impact of Intracranial Pressure Monitoring on Prognosis of Patients With Severe Traumatic Brain Injury: A PRISMA Systematic Review and Meta-Analysis. Medicine (Baltimore) 2016;95:e2827. [Crossref] [PubMed]
- Shibahashi K, Ohbe H, Matsui H, et al. Real-world benefit of intracranial pressure monitoring in the management of severe traumatic brain injury: a propensity score matching analysis using a nationwide inpatient database. J Neurosurg 2023;139:1514-22. [Crossref] [PubMed]
- Shen L, Wang Z, Su Z, et al. Effects of Intracranial Pressure Monitoring on Mortality in Patients with Severe Traumatic Brain Injury: A Meta-Analysis. PLoS One 2016;11:e0168901. [Crossref] [PubMed]
- Paek SC, Meemon N, Wan TT. Thailand's universal coverage scheme and its impact on health-seeking behavior. Springerplus 2016;5:1952. [Crossref] [PubMed]
- National Health Security Office. The way forward of Thai UHC: Address quality and safe care, social determinants of health, primary care, and multi-sectoral collaboration. 2014 [cited 2023 Jul 5]. Available online: https://www.nhso.go.th/storage/files/shares/PDF2/fund_medi05.pdf
- Agrawal D, Raghavendran K, Schaubel DE, et al. A Propensity Score Analysis of the Impact of Invasive Intracranial Pressure Monitoring on Outcomes after Severe Traumatic Brain Injury. J Neurotrauma 2016;33:853-8. [Crossref] [PubMed]
- Tunthanathip T, Oearsakul T. Application of machine learning to predict the outcome of pediatric traumatic brain injury. Chin J Traumatol 2021;24:350-5. [Crossref] [PubMed]
- Vieira RC, Paiva WS, de Oliveira DV, et al. Diffuse Axonal Injury: Epidemiology, Outcome and Associated Risk Factors. Front Neurol 2016;7:178. [Crossref] [PubMed]
- Brain Trauma Foundation. J Neurotrauma 2007;24:S55-8. [Crossref] [PubMed]
- Zhang Z, Kim HJ, Lonjon G, et al. Balance diagnostics after propensity score matching. Ann Transl Med 2019;7:16. [Crossref] [PubMed]
- Zhao QY, Luo JC, Su Y, et al. Propensity score matching with R: conventional methods and new features. Ann Transl Med 2021;9:812. [Crossref] [PubMed]
- Chowdhury MZI, Turin TC. Variable selection strategies and its importance in clinical prediction modelling. Fam Med Community Health 2020;8:e000262. [Crossref] [PubMed]
- Sekhon JS, Saarinen T. Package ‘MatchIt’. 2022 [cited 2023 Jul 5]. Available online: https://cran.r-project.org/web/packages/Matching/Matching.pdf
- Abu Hamdeh S, Marklund N, Lewén A, et al. Intracranial pressure elevations in diffuse axonal injury: association with non-hemorrhagic MR lesions in central mesencephalic structures. J Neurosurg 2018;131:604-11. [Crossref] [PubMed]
- Stocchetti N, Picetti E, Berardino M, et al. Clinical applications of intracranial pressure monitoring in traumatic brain injury: report of the Milan consensus conference. Acta Neurochir (Wien) 2014;156:1615-22. [Crossref] [PubMed]
- Hellewell SC, Ziebell JM, Lifshitz J, et al. Impact Acceleration Model of Diffuse Traumatic Brain Injury. Methods Mol Biol 2016;1462:253-66. [Crossref] [PubMed]
- Zeiler FA, Mathieu F, Monteiro M, et al. Diffuse Intracranial Injury Patterns Are Associated with Impaired Cerebrovascular Reactivity in Adult Traumatic Brain Injury: A CENTER-TBI Validation Study. J Neurotrauma 2020;37:1597-608. [Crossref] [PubMed]
- Jha SK. Cerebral Edema and its Management. Med J Armed Forces India 2003;59:326-31. [Crossref] [PubMed]
- Yuan Q, Wu X, Sun Y, et al. Impact of intracranial pressure monitoring on mortality in patients with traumatic brain injury: a systematic review and meta-analysis. J Neurosurg 2015;122:574-87. [Crossref] [PubMed]
- Khormi YH, Senthilselvan A, O'kelly C, et al. Adherence to brain trauma foundation guidelines for intracranial pressure monitoring in severe traumatic brain injury and the effect on outcome: A population-based study. Surg Neurol Int 2020;11:118. [Crossref] [PubMed]
- Sahjpaul R, Girotti M. Intracranial pressure monitoring in severe traumatic brain injury--results of a Canadian survey. Can J Neurol Sci 2000;27:143-7. [Crossref] [PubMed]
- Biersteker HA, Andriessen TM, Horn J, et al. Factors influencing intracranial pressure monitoring guideline compliance and outcome after severe traumatic brain injury. Crit Care Med 2012;40:1914-22. [Crossref] [PubMed]
- Mauritz W, Steltzer H, Bauer P, et al. Monitoring of intracranial pressure in patients with severe traumatic brain injury: an Austrian prospective multicenter study. Intensive Care Med 2008;34:1208-15. [Crossref] [PubMed]
- Chesnut RM, Temkin N, Carney N, et al. A trial of intracranial-pressure monitoring in traumatic brain injury. N Engl J Med 2012;367:2471-81. [Crossref] [PubMed]
- Chesnut RM, Temkin N, Videtta W, et al. Consensus-Based Management Protocol (CREVICE Protocol) for the Treatment of Severe Traumatic Brain Injury Based on Imaging and Clinical Examination for Use When Intracranial Pressure Monitoring Is Not Employed. J Neurotrauma 2020;37:1291-9. [Crossref] [PubMed]
- Chesnut RM, Temkin N, Videtta W, et al. Testing the Impact of Protocolized Care of Patients With Severe Traumatic Brain Injury Without Intracranial Pressure Monitoring: The Imaging and Clinical Examination Protocol. Neurosurgery 2023;92:472-80. [Crossref] [PubMed]
- Bafeta A, Dechartres A, Trinquart L, et al. Impact of single centre status on estimates of intervention effects in trials with continuous outcomes: meta-epidemiological study. BMJ 2012;344:e813. [Crossref] [PubMed]
- Taweesomboonyat C, Kaewborisutsakul A, Tunthanathip T, et al. Necessity of in-hospital neurological observation for mild traumatic brain injury patients with negative computed tomography brain scans. J Health Sci Med Res 2020;38:267-74. [Crossref]
- Fuangworawong P, LaGrone LN, Chadbunchachai W, et al. Assessment of trauma quality improvement activities at public hospitals in Thailand. Int J Surg 2016;33 Pt A:88-95.
- Pirracchio R, Resche-Rigon M, Chevret S. Evaluation of the propensity score methods for estimating marginal odds ratios in case of small sample size. BMC Med Res Methodol 2012;12:70. [Crossref] [PubMed]
- Yousefi M, Assari Arani A, Sahabi B, et al. Household Health Costs: Direct, Indirect and Intangible. Iran J Public Health 2014;43:202-9. [PubMed]
Cite this article as: Jitchanvichai J, Tunthanathip T. Effect of intracranial pressure monitoring on mortality following severe traumatic brain injury in Thailand: propensity score matching methods. J Emerg Crit Care Med 2024;8:1.