Timing of intubation and clinical outcomes in critically ill COVID-19 patients with respiratory failure
Highlight box
Key findings
• Deferred intubation (DI) with non-invasive respiratory support is feasible for coronavirus disease 2019 (COVID-19) patients with respiratory failure, provided careful patient selection and close monitoring.
• In cases of severe respiratory failure or clinical deterioration, prompt intubation remains crucial to prevent adverse outcomes.
What is known and what is new?
• Recent studies have countered the practice of early intubation (EI) in COVID-19 patients, stating that it fails to correlate with a decrease in intensive care unit (ICU) length of stay or enhance survival rates. Instead, studies have highlighted the significance of employing non-invasive respiratory support in the management of COVID-19 patients experiencing acute hypoxemic respiratory failure. However, the impact of the timing of intubation in COVID-19 patients remains unclear.
• Our study showed that, in contrast to DI, EI was associated with fewer ICU and ventilator-free days, along with an increased rate of secondary bacterial infections. However, when compared to late intubation (patients who failed non-invasive respiratory support), EI showed higher ICU and ventilator-free days, and reduced hospital length of stay.
What is the implication, and what should change now?
• The non-invasive respiratory support can be employed in COVID-19 patients experiencing respiratory failure, but timely intubation is crucial when clinical deterioration occurs to avoid adverse patient outcomes.
Introduction
Coronavirus disease 2019 (COVID-19) is an infectious disease caused by the severe acute respiratory syndrome coronavirus 2 (SARS-CoV-2) virus. The World Health Organization (WHO) declared the COVID-19 outbreak a pandemic on March 11th, 2020, due to its rapid global spread. According to the data from WHO (https://data.who.int/dashboards/covid19; Accessed January 30, 2024), the global count for confirmed COVID-19 cases has reached 774 million, with over 7 million reported deaths.
COVID-19 patients can present with a wide range of respiratory manifestations, varying from upper respiratory infection symptoms to acute respiratory distress syndrome (ARDS). While most patients experience mild to moderate illness, a considerable proportion of patients suffer from serious illness and death. A study from China reported that almost 20% of the infected cases experienced hypoxic respiratory failure and more than a quarter of patients needed intensive care unit (ICU) admission (1,2).
Early in the pandemic of COVID-19, the management of COVID-19-associated ARDS (CARDS) was approached in the same way as general ARDS including early intubation (EI) to prevent patient self-inflicted lung injury (P-SILI) and to reduce mortality (3,4). However, CARDS differs from general ARDS as it has distinct disease phenotypes which can lead to heterogeneity of response to therapeutic interventions (5). The American Thoracic Society’s 2024 update on the management of adult ARDS patients recommends considering the ratio of partial pressure arterial oxygen (PaO2) and fraction of inspired oxygen (FiO2) when establishing respiratory support strategies (6). Nevertheless, the optimal timing of intubation for critically ill COVID-19 patients with acute hypoxic respiratory failure remains a subject of considerable debate. Several studies have argued against EI in COVID-19 patients, stating that EI is neither associated with a reduction of ICU length of stay nor improves survival outcomes (7,8). Previous evidence and the European Society of Intensive Care Medicine (ESICM) on ARDS 2023 guideline have indicated that treatment with high-flow nasal cannula (HFNC) or non-invasive ventilation (NIV) can play a vital role in managing patients with moderate to severe acute hypoxemic respiratory failure caused by COVID-19 (9-11). These strategies can improve ventilatory efficiency, reduce work of breathing, and be easily applied to patients who are not admitted to the ICU. Non-intubation strategies such as HFNC or NIV may have alleviated the reported crisis of ventilator and ICU shortages during the early months of the pandemic.
The timing of intubation in COVID-19 patients varies among studies. Some studies reported that the median time from admission to intubation ranged from 1 to 3 days (12,13). The variation in the time taken to initiate invasive mechanical ventilation can significantly influence clinical outcomes (12,14,15). However, the effects of intubation timing on clinical outcomes are still unclear, and there is a lack of evidence on this matter. This study aimed to evaluate the impact of EI and deferred intubation (DI) on ICU-free days in critically ill COVID-19 patients. We present this article in accordance with the STROBE reporting checklist (available at https://jeccm.amegroups.com/article/view/10.21037/jeccm-24-47/rc).
Methods
Study setting and population
This retrospective cohort study was carried out at the King Chulalongkorn Memorial Hospital, a tertiary hospital in Bangkok, Thailand, from April 1st to December 31st, 2021. All subjects who were 18 years old or over with laboratory-confirmed SARS-CoV-2 infection and suffered from respiratory failure requiring ICU admission were enrolled. Patients with combined diagnosis of bacterial and fungal pneumonia or those receiving palliative care were excluded from the study. Participant data was collected from electronic medical records in a deidentified manner. Baseline characteristics (e.g., age, gender, and body mass index), Acute Physiology and Chronic Health Evaluation II (APACHE II) score, oxygen saturation (SpO2) to FiO2 (SF) ratio, respiratory rate oxygenation (ROX) index (SF ratio/respiratory rate), medications (e.g., antiviral agents, immunosuppressants, and inotropes/vasopressors), and laboratory results were recorded (16). APACHE II score, SF ratio, ROX index, and laboratory results were recorded on the first day of ICU admission.
The study was conducted in accordance with the Declaration of Helsinki (as revised in 2013). The study was approved by the Institutional Review Board of the Faculty of Medicine, Chulalongkorn University (IRB No. 1030/64) and registered in the Thai Clinical Trials Registry (TCTR20220328006). Individual consent for this retrospective analysis was waived.
EI and DI
The subjects were classified into EI and DI groups. The EI group was defined as intubation within the first 72 hours of ICU admission. The DI group was treated with non-invasive respiratory support including HFNC or NIV. In the DI group, the subjects who failed with the non-invasive respiratory support were subsequently intubated and further categorized into the late intubation (LI) group (intubation after 72 hours of ICU admission), while the subjects who succeeded and had no need for intubation were categorized into the HFNC/NIV group. Primary physicians considered the type and setting of respiratory support as they deemed appropriate.
Study outcomes
The primary outcome was ICU-free days within the first 30 days of ICU admission. The ICU-free days were defined as 30 minus the number of days in the ICU and assigned 0 ICU-free days for death or admission in the ICU for longer than 30 days. The secondary outcomes were 30-day mortality, ventilator-free days within 30 days, hospital length of stay, and secondary bacterial infections during ICU admission. Thirty-day mortality included mortality from all causes. Ventilator-free days were defined as 30 minus the number of intubation days and assigned as 30 for the HFNC/NIV group. Secondary bacterial infections were identified when there was evidence of positive bacterial culture in blood, sputum, or urine during the ICU admission. The exploratory outcome was simplified risk factors of failure in non-invasive respiratory support. A prespecified subgroup analysis comparing outcomes between the EI group and LI groups (excluding subjects with successful non-invasive respiratory support) was performed to reduce the disparity in severity of the illness between the groups.
Statistical analysis
Continuous data with normal distribution was reported as mean with standard deviation (SD) and compared by using the unpaired t-test. Non-normally distributed data was reported as median with interquartile range (IQR) and compared by using the Mann-Whitney U test. Categorical data were reported as frequency with percentage and compared by using the chi-square test. The Kaplan-Meier survival analysis and log-rank test were used to compare successful ICU discharge and mortality between groups. The Cox proportional hazards model was utilized to estimate the hazard ratio (HR) of successful ICU discharge and mortality. Potentially associated variables with P<0.1 in the univariate model were adjusted in the multivariate model. Logistic regression analysis was used to identify risk factors for failure of using non-invasive respiratory support. Multivariable logistic regression analysis included variables with P<0.1 from the univariate analysis. A two-tailed P of less than 0.05 was considered statistically significance. Stata version 17 (StataCorp, College Station, TX, USA) was used for statistical analysis.
Results
There were 422 subjects enrolled in the study period. Twenty-five subjects were excluded as they met exclusion criteria, leaving 397 subjects included in the final analysis: 102 subjects (25.7%) in the EI group, and 295 subjects (74.3%) in the DI group. The mean age was 61.7 years old and 58.2% were men. In the DI group, 194 subjects (65.8%) achieved successful treatment with HFNC or NIV (Figure 1). ARDS was diagnosed in 129 subjects (32.5%) at the time of enrollment. Median APACHE II score, SF ratio, and ROX index at baseline were 11 [7, 16], 168 [125, 245], and 7.75 [4.84, 10.53], respectively. Septic shock was present in 107 (27.0%) subjects. Most of the patients received corticosteroids (96.7%) and antiviral drugs including favipiravir (75.6%) and remdesivir (81.9%). Detailed baseline characteristics and laboratory data are presented in Table 1 and Table S1.
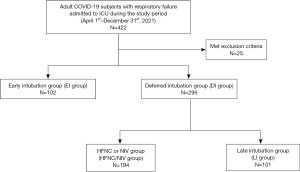
Table 1
Characteristics | Total (n=397) | EI group (n=102) | DI group | P value (EI vs. DI) | P value (EI vs. LI) | ||
---|---|---|---|---|---|---|---|
Total (n=295) | HFNC/NIV group (n=194) | LI group (n=101) | |||||
Age (years old) | 61.7±16.2 | 60.4±14.5 | 62.2±16.8 | 61.3±17.7 | 63.9±1.5 | 0.34 | 0.09 |
Age ≥65 years old | 179 (45.1) | 39 (38.2) | 140 (47.5) | 84 (43.3) | 56 (55.4) | 0.11 | 0.01 |
Male | 231 (58.2) | 58 (56.9) | 173 (58.6) | 112 (57.7) | 61 (60.4) | 0.75 | 0.61 |
BMI (kg/m2) | 26.9±6.9 | 28.1±7.66 | 26.6±6.6 | 26.5±6.3 | 26.3±0.7 | 0.05 | 0.09 |
BMI ≥30 kg/m2 | 93 (23.4) | 31 (30.4) | 62 (21.0) | 42 (21.6) | 20 (19.8) | 0.05 | 0.08 |
ARDS | 129 (32.5) | 51 (50.0) | 78 (26.4) | 18 (9.3) | 60 (59.4) | <0.001* | 0.18 |
Septic shock | 107 (27.0) | 46 (45.1) | 61 (20.7) | 12 (6.2) | 49 (48.5) | <0.001* | 0.62 |
APACHE II score | 11 [7, 16] | 13 [9, 18] | 10 [7, 15] | 9 [6, 13] | 14 [10, 19] | <0.001* | 0.24 |
SF ratio | 168 [125, 245] | 160 [116, 243] | 190 [133, 245] | 226 [163, 281] | 123 [100, 170] | 0.12 | 0.001 |
ROX index | 7.75 [4.84, 10.53] | 6.43 [3.85, 9.8] | 7.92 [5.39, 10.88] | 9.43 [7.31, 11.98] | 4.84 [3.81, 7.58] | 0.003* | 0.04 |
ROX index <4.88 | 100 (25.2) | 38 (37.3) | 62 (21.0) | 11 (5.7) | 51 (50.5) | 0.001 | 0.06 |
Antiviral drugs | |||||||
Favipiravir | 300 (75.6) | 53 (52.0) | 247 (83.7) | 157 (80.9) | 90 (89.1) | <0.001* | <0.001* |
Remdesivir | 325 (81.9) | 88 (86.3) | 237 (80.3) | 155 (79.9) | 82 (81.2) | 0.18 | 0.33 |
Immunosuppressive drugs | |||||||
Corticosteroids | 384 (96.7) | 98 (96.1) | 286 (96.9) | 187 (96.4) | 99 (98.0) | 0.67 | 0.41 |
Tocilizumab | 121 (30.5) | 36 (35.3) | 85 (28.8) | 50 (25.8) | 35 (34.7) | 0.22 | 0.92 |
Prone position | <0.001* | 0.09 | |||||
No | 205 (51.6) | 58 (56.9) | 147 (49.8) | 103 (53.1) | 44 (43.6) | ||
Awake prone | 141 (35.5) | 19 (18.6) | 122 (41.4) | 91 (46.9) | 31 (30.7) | ||
Prone with paralysis | 51 (12.8) | 25 (24.5) | 26 (8.8) | 0 | 26 (25.7) | ||
Inotropes/vasopressors | 162 (40.8) | 75 (73.5) | 87 (29.5) | 21 (10.8) | 66 (65.3) | <0.001* | 0.21 |
Lactate (mmol/L) | 1.5 [1.1, 2.3] | 1.6 [1.1, 2.6] | 1.5 [1.1, 2.1] | 1.3 [1, 1.9] | 1.7 [1.3, 2.4] | 0.06 | 0.85 |
Lactate ≥2 mmol/L | 104 (26.2) | 38 (37.3) | 66 (22.4) | 30 (15.5) | 36 (35.6) | 0.65 | 0.81 |
Data are presented as mean ± SD, n (%), or median [IQR]. *, P<0.05. EI, early intubation; DI, deferred intubation; HFNC, high-flow nasal cannula; NIV, non-invasive ventilation; LI, late intubation; BMI, body mass index; ARDS, acute respiratory distress syndrome; APACHE II, acute physiology and chronic health evaluation II; ROX, respiratory rate oxygenation; IQR, interquartile range.
The EI group exhibited a significantly higher prevalence of ARDS (50.0% EI vs. 26.4% DI, P<0.001) and septic shock (45.1% EI vs. 20.7% DI group, P<0.001). Comparisons of other key indices revealed that the EI group had a higher APACHE II score {13 [9, 18] EI vs. 10 [7, 15] DI, P<0.001}, lower ROX index {6.43 [3.85, 9.8] EI vs. 7.92 [5.39, 10.88] DI, P=0.003}, and a greater need for inotropes/vasopressors (73.5% EI vs. 29.5% DI, P<0.001).
Primary and secondary outcomes
The EI group had a significantly lower rate of successful ICU discharge with a median ICU-free days of 4 [0, 20] days compared to 19 [0, 25] days in the DI group (P<0.001). The 30-day mortality rate was also higher in the EI group (29.4% EI vs. 15.3% DI, P=0.002). Ventilator-free days were lower in the EI group with a median of 8 [0, 22] days compared to 30 [16, 30] days in the DI group. The rate of secondary bacterial infections was higher in the EI group (56.9% EI vs. 40.3% DI, P=0.004). The hospital length of stay was not statistically different between the EI and DI groups {22 [15, 36] vs. 21 [14, 33] days, respectively, P=0.46} (Table 2).
Table 2
Outcomes | Total (n=397) | EI group (n=102) | DI group | P value (EI vs. DI) | P value (EI vs. LI) | ||
---|---|---|---|---|---|---|---|
Total (n=295) | HFNC/NIV group (n=194) | LI group (n=101) | |||||
ICU-free days | 15 [0, 24] | 4 [0, 20] | 19 [0, 25] | 24 [15, 27] | 0 [0, 13] | <0.001* | 0.04* |
30-day all-cause mortality | 75 (18.9) | 30 (29.4) | 45 (15.3) | 18 (9.3) | 27 (26.7) | 0.002* | 0.67 |
Ventilator-free days | 28 [0, 30] | 8 [0, 22] | 30 [16, 30] | 30 [30, 30] | 0 [0, 17] | <0.001* | 0.03* |
Hospital length of stays (days) | 21 [14, 33] | 22 [15, 36] | 21 [14, 33] | 16 [14, 24] | 33 [22, 47] | 0.46 | <0.001* |
Secondary bacterial infections | 177 (44.6) | 58 (56.9) | 119 (40.3) | 46 (23.7) | 73 (72.3) | 0.004* | 0.02* |
Data are presented as median [IQR] or n (%). *, P<0.05. EI, early intubation; DI, deferred intubation; HFNC, high-flow nasal cannula; NIV, non-invasive ventilation; LI, late intubation; ICU, intensive care unit; IQR, interquartile range.
When compared to the LI group, the EI group had significantly higher ICU-free days {4 [0, 20] EI vs. 0 [0, 13] LI, P=0.04} and ventilator-free days {8 [0, 22] EI vs. 0 [0, 17] LI, P=0.03}. The subjects in the EI group also had a significantly lower hospital length of stay {22 [15, 36] EI vs. 33 [22, 47] LI, P<0.001} and rate of secondary bacterial infections (56.9% EI vs. 72.3% LI, P=0.02). The 30-day mortality rate was not statistically different between the EI and LI groups (29.4% vs. 26.7%, respectively, P=0.67) (Table 2).
Survival analysis
The cumulative probability of successful ICU discharge was lower in the EI group with a log-rank P value of 0.002 (Figure 2A). The 30-day mortality rate was also higher in the EI group with a log-rank P value of <0.001 (Figure 2B). The EI group was associated with a lower rate of successful ICU discharge [HR, 0.63; 95% confident interval (CI): 0.46–0.85; P=0.003] in the univariable Cox proportional hazards model. However, these associations lost significance after adjusting for potential confounding factors [adjusted HR (aHR), 1.08; 95% CI: 0.78–1.50; P=0.64]. In the multivariable Cox proportional hazards model, a higher age, higher APACHE II score, treated with prone positioning and paralysis, and received inotropes or vasopressors were associated with a lower rate of successful ICU discharges. In addition, higher age, higher APACHE II score, and receipt of inotropes or vasopressors were associated with a higher 30-day mortality (Table 3).
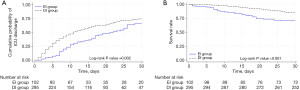
Table 3
Parameters | Successful ICU discharge | Mortality | |||||||
---|---|---|---|---|---|---|---|---|---|
HR† (95% CI) | P value§ | aHR† (95% CI) | P value¶ | HR‡ (95% CI) | P value§ | aHR‡ (95% CI) | P value¶ | ||
EI vs. DI | 0.63 (0.46–0.85) | 0.003 | 1.08 (0.78–1.50) | 0.64 | 2.21 (1.39–3.52) | 0.001 | 1.48 (0.86–2.44) | 0.16 | |
Age | 0.98 (0.97–0.98) | <0.001 | 0.99 (0.98–0.99) | 0.001 | 1.04 (1.02–1.06) | <0.001 | 1.02 (1.01–1.05) | 0.007 | |
Male | 0.80 (0.63–1.03) | 0.08 | 0.99 (0.76–1.27) | 0.91 | 1.15 (0.72–1.84) | 0.56 | |||
BMI | 1.01 (0.999–1.03) | 0.07 | 1.00 (0.98–1.02) | 0.85 | 0.93 (0.89–0.97) | 0.001 | 0.97 (0.93–1.02) | 0.22 | |
ARDS | 0.44 (0.33–0.59) | <0.001 | 0.77 (0.56–1.06) | 0.11 | 1.46 (0.92–2.31) | 0.11 | |||
Septic shock | 0.29 (0.21–0.41) | <0.001 | 0.84 (0.49–1.44) | 0.52 | 1.50 (0.93–2.41) | 0.10 | 0.43 (0.25–0.75) | 0.003 | |
APACHE II | 0.90 (0.88–0.92) | <0.001 | 0.94 (0.91–0.96) | <0.001 | 1.15 (1.11–1.18) | <0.001 | 1.10 (1.06–1.13) | <0.001 | |
Antiviral drugs | |||||||||
Favipiravir | 1.00 (0.99–1.01) | 0.97 | 0.75 (0.45–1.25) | 0.27 | |||||
Remdesivir | 0.79 (0.58–1.08) | 0.14 | 0.97 (0.54–1.73) | 0.92 | |||||
Immunosuppressive drugs | |||||||||
Corticosteroid | 0.80 (0.39–1.61) | 0.53 | 0.53 (0.19–1.45) | 0.21 | |||||
Tocilizumab | 0.82 (0.62–1.07) | 0.14 | 0.80 (0.48–1.34) | 0.40 | |||||
Prone positioning | |||||||||
Awake prone | 1.07 (0.83–1.39) | 0.58 | 0.77 (0.59–1.01) | 0.06 | 0.51 (0.30–0.88) | 0.02 | 0.79 (0.45–1.39) | 0.42 | |
Prone with paralysis | 0.29 (0.17–0.48) | <0.001 | 0.38 (0.22–0.66) | 0.001 | 0.72 (0.36–1.48) | 0.37 | 0.66 (0.32–1.37) | 0.27 | |
Inotropes/vasopressors | 0.26 (0.19–0.35) | <0.001 | 0.41 (0.26–0.66) | <0.001 | 3.88 (2.38–6.35) | <0.001 | 3.96 (2.11–7.46) | <0.001 |
†, HR <1.0 means less ICU-free days; ‡, HR >1.0 means a higher mortality rate; §, P value for HR (95% CI); ¶, P value for aHR (95% CI). ICU, intensive care unit; EI, early intubation; DI, deferred intubation; HR, hazard ratio; CI, confident interval; aHR, adjusted hazard ratio; BMI, body mass index; ARDS, acute respiratory distress syndrome; APACHE II, acute physiology and chronic health evaluation II.
In the subgroup analysis between the EI and LI groups, the cumulative probability of successful ICU discharge was higher in the EI group with a log-rank P value of 0.002, although the difference in the cumulative incidence of 30-day mortality was not statistically significant (log-rank P value of 0.46) (Figure 3A,3B). The EI group was associated with a higher rate of successful ICU discharge in both univariable and multivariable Cox proportional hazard models (aHR, 1.81; 95% CI: 1.18–2.79; P=0.007). A difference in mortality between the groups was also not observed (HR, 1.21; 95% CI: 0.72–2.04; P=0.47) (Table S2).
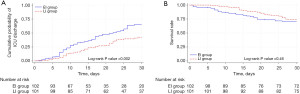
Failure of non-invasive respiratory support
A total of 101 subjects (34.2%) in the DI group experienced failure in non-invasive respiratory support. After adjusting for potential confounding factors, the presence of ARDS [adjusted odds ratio (aOR), 9.0; 95% CI: 4.2–19.2; P<0.001], septic shock (aOR, 5.4; 95% CI: 2.3–12.6; P<0.001), ROX index of less than 4.88 (aOR, 9.6; 95% CI: 4.0–23.3; P<0.001), and a lactate level of 2 mmol/L or more (aOR, 3.4; 95% CI: 1.5–7.5; P<0.001) were associated with failure of non-invasive respiratory support (Table 4).
Table 4
Variables | OR (95% CI) | P value† | aOR (95% CI) | P value‡ |
---|---|---|---|---|
Age ≥65 years old | 1.6 (1.0–2.7) | 0.048* | 1.8 (0.9–3.5) | 0.11 |
BMI ≥30 kg/m2 | 0.9 (0.5–1.6) | 0.71 | ||
ARDS | 14.3 (7.6–26.8) | <0.001* | 9.0 (4.2–19.2) | <0.001* |
Septic shock | 14.3 (7.1–28.9) | <0.001* | 5.4 (2.3–12.6) | <0.001* |
ROX index <4.88 | 17 (8.2–35.0) | <0.001* | 9.6 (4.0–23.3) | <0.001* |
Lactate ≥2 mmol/L | 3.0 (1.7–5.3) | <0.001* | 3.4 (1.5–7.5) | <0.001* |
†, P value for OR (95% CI); ‡, P value for aOR (95% CI); *, P<0.05. OR, odds ratio; CI, confident interval; aOR, adjusted odds ratio; BMI, body mass index; ARDS, acute respiratory distress syndrome; ROX, respiratory rate oxygenation.
Discussion
Acute hypoxic respiratory failure is the most common clinical presentation in critically ill COVID-19 patients and up to 30% of these patients develop CARDS (17). Respiratory support is an important strategy leading to the successful treatment of respiratory failure in COVID-19 patients. An early endotracheal intubation was recommended in the very early stages of the pandemic due to the severity of the disease and concerns about aerosol spreading from high-flow oxygen supplementation of more than 6 L/min (18). However, a study from Wuhan in 2020 revealed that EI was associated with a higher mortality attributed to inadequate staff and resources at that time (19). Subsequently, several studies demonstrated the benefit of using non-invasive respiratory support such as HFNC and NIV in preventing intubation and reducing mortality (20-24). Utilizing non-invasive respiratory support is preferable to conventional oxygen therapy as it has the potential to decrease respiratory effort resulting in a lower risk of P-SILI. Furthermore, avoiding intubation can lessen its complications including delirium, muscle weakness, and ventilator-associated pneumonia (25). This retrospective cohort study demonstrated that the EI group had a lower rate of successful ICU discharges with a median ICU-free days of 4 [0, 20] days compared to 19 [0, 25] days in the DI group, P<0.001. The 30-day mortality and secondary bacterial infection rates were higher in the EI group (29.4% vs. 15.3%, P=0.002 and 56.9% vs. 40.3%, P=0.004, respectively). However, the baseline characteristics of the EI group were worse than the DI group resulting in an unavoidable treatment bias. For example, patients with more severe respiratory failure were prone to be selected for EI. Therefore, the outcomes of this study may not only depend on the treatment strategies but also be affected by the baseline disease severity. This conclusion was supported by the survival analysis with the multivariable Cox proportional hazards model. After adjusting with potential confounding factors that mostly indicated the disease severity (e.g., age, presence of ARDS, septic shock, APACHE II score, and being treated with inotropes/vasopressors), EI was not associated with ICU discharge or mortality. Furthermore, some of the disease severity indicators showed significant association with patient outcomes.
A prespecified subgroup analysis between the EI and LI groups was performed to address this bias issue. Among 295 subjects in the DI group, 101 (34.2%) subjects who failed to respond to non-invasive respiratory support and were subsequently intubated were categorized into the LI group. Baseline characteristics between the EI and LI groups were similar in terms of disease severity. Surprisingly, subgroup analysis results were different from the main analysis. Compared to the LI group, the EI group had more ICU-free days and ventilator-free days and a shorter hospital length of stay, and a lower rate of secondary bacterial infection. However, the 30-day mortality rate was not statistically significant different. Supported by the results of the Cox proportional hazards model, EI was associated with a higher rate of successful ICU discharge compared to the LI group (aHR, 1.81; 95% CI: 1.18–2.79; P=0.007), but not with a 30-day mortality rate after adjusting for potential confounding factors. Hence, the essential part of treating respiratory failure in COVID-19 patients may not be the strategy of EI or DI itself, but the proper patient selection for these strategies. An inappropriate use of non-invasive respiratory support (e.g., patients with severe respiratory failure) can cause delayed intubation leading to P-SILI and worse outcomes (26). P-SILI is a contributing factor that exacerbates a patient’s clinical condition, resulting in increased lung injury and mortality rates along with reduced ICU-free days and ventilator-free days (27,28). The worst outcomes in the LI group may be explained by the consequences of P-SILI.
Identifying the risk factors of failure in non-invasive respiratory support is crucial to avoid inappropriate patient selection for DI. This study demonstrated that the presence of ARDS (aOR, 9.0; 95% CI: 4.2–19.2; P<0.001), septic shock (aOR, 5.4; 95% CI: 2.3–12.6; P<0.001), ROX index of less than 4.88 (aOR, 9.6; 95% CI: 4.0–23.3; P<0.001), and a lactate level of 2 mmol/L or more (aOR, 3.4; 95% CI: 1.5–7.5; P<0.001) were associated with failure of non-invasive respiratory support. The ROX index has been studied and validated in acute hypoxemic respiratory failure and COVID-19 with acute respiratory failure in numerous studies (29-31). ARDS, shock, and high Sequential Organ Failure Assessment (SOFA) scores were also found to be associated with the failure of non-invasive respiratory support (32-34). These four factors can be easily assessed in routine clinical practice and may be utilized as additional markers when considering EI, particularly in the situation of an overcrowded healthcare system.
The current study suggested that COVID-19 patients with respiratory failure can be given a trial on HFNC or NIV after careful evaluation for the risk of non-invasive respiratory support failure. In addition, close observation and early detection of worsening respiratory conditions are also mandatory. If the indication for intubation arises, a physician should promptly intubate without delay. EI did show benefits over LI and should be considered in patients with severe respiratory failure due to higher ICU-free days and ventilator-free days along with reduced rate of secondary bacterial infection and length of hospital stays. The increase in ICU-free days and ventilator-free days can significantly improve resource utilization, especially during the pandemic. ICU beds and ventilators are typically a limited resource even at large tertiary hospitals. The optimal time and patient selection for intubation are crucial, not only for better patient outcomes but also for improved resource management of healthcare systems.
This study had several limitations that need to be addressed. Firstly, differences in baseline disease severity and clinician treatment selection between the groups may cause treatment bias and affect outcomes of interest. Multivariable Cox proportional hazards model adjusted for potential confounding factors and prespecified subgroup analysis were performed to minimize this limitation. These analyses revealed conformable results making the study’s findings more robust. Secondly, the COVID-19 vaccines were insufficient during the study period and some participants might not have been vaccinated. In addition, the lack of vaccine data on each participant may bias results. Due to these limitations, generalizing the study results may not be prudent. However, a study in 2022 found that vaccination reduced mortality in COVID-19 patients with CARDS who were intubated but did not demonstrate any differences in ICU-free days (35). Thirdly, although our study identified the risk factors of failure in non-invasive respiratory support, this study was not designed to test the performance of these factors. Future studies should focus on validating these risk factors and reassessing the effect of EI and DI in the vaccinated population. Lastly, cycle threshold (Ct) value of SARS-CoV-2 real-time reverse transcriptase-polymerase chain reaction (RT-PCR) assays could not be analyzed due to the lack of a standardized protocol for specimen collection timing relative to disease course. Additionally, data on the intubation period like procedure time, sedative agents, neuromuscular blockage agents, and presence of cardiovascular collapse during intubation were unavailable. These factors could potentially influence clinical outcomes. Thus, future planned or protocolized prospective study design is needed.
Conclusions
This study demonstrated that DI with non-invasive respiratory support (e.g., HFNC and NIV) can be utilized in COVID-19 patients with respiratory failure employing careful patient selection and close monitoring. In patients with severe respiratory failure or clinical worsening on observation, prompt intubation remains the key clinical intervention to avoid adverse outcomes.
Acknowledgments
The authors would like to thank all the healthcare professionals who provided care to COVID-19 patients throughout the pandemic. The language of this manuscript was improved by the English editing service, Research Affairs, Faculty of Medicine, Chulalongkorn University.
Funding: None.
Footnote
Reporting Checklist: The authors have completed the STROBE reporting checklist. Available at https://jeccm.amegroups.com/article/view/10.21037/jeccm-24-47/rc
Data Sharing Statement: Available at https://jeccm.amegroups.com/article/view/10.21037/jeccm-24-47/dss
Peer Review File: Available at https://jeccm.amegroups.com/article/view/10.21037/jeccm-24-47/prf
Conflicts of Interest: All authors have completed the ICMJE uniform disclosure form (available at https://jeccm.amegroups.com/article/view/10.21037/jeccm-24-47/coif). The authors have no conflicts of interest to declare.
Ethical Statement: The authors are accountable for all aspects of the work in ensuring that questions related to the accuracy or integrity of any part of the work are appropriately investigated and resolved. The study was conducted in accordance with the Declaration of Helsinki (as revised in 2013). The study was approved by the Institutional Review Board of the Faculty of Medicine, Chulalongkorn University (IRB No. 1030/64) and registered in the Thai Clinical Trials Registry (TCTR20220328006). Individual consent for this retrospective analysis was waived.
Open Access Statement: This is an Open Access article distributed in accordance with the Creative Commons Attribution-NonCommercial-NoDerivs 4.0 International License (CC BY-NC-ND 4.0), which permits the non-commercial replication and distribution of the article with the strict proviso that no changes or edits are made and the original work is properly cited (including links to both the formal publication through the relevant DOI and the license). See: https://creativecommons.org/licenses/by-nc-nd/4.0/.
References
- Wu C, Chen X, Cai Y, et al. Risk Factors Associated With Acute Respiratory Distress Syndrome and Death in Patients With Coronavirus Disease 2019 Pneumonia in Wuhan, China. JAMA Intern Med 2020;180:934-43. [Crossref] [PubMed]
- Wu Z, McGoogan JM. Characteristics of and Important Lessons From the Coronavirus Disease 2019 (COVID-19) Outbreak in China: Summary of a Report of 72 314 Cases From the Chinese Center for Disease Control and Prevention. JAMA 2020;323:1239-42. [Crossref] [PubMed]
- Saguil A, Fargo MV. Acute Respiratory Distress Syndrome: Diagnosis and Management. Am Fam Physician 2020;101:730-8. [PubMed]
- Kangelaris KN, Ware LB, Wang CY, et al. Timing of Intubation and Clinical Outcomes in Adults With Acute Respiratory Distress Syndrome. Crit Care Med 2016;44:120-9. [Crossref] [PubMed]
- Lascarrou JB. COVID-19-related ARDS: one disease, two trajectories, and several unanswered questions. Lancet Respir Med 2021;9:1345-7. [Crossref] [PubMed]
- Qadir N, Sahetya S, Munshi L, et al. An Update on Management of Adult Patients with Acute Respiratory Distress Syndrome: An Official American Thoracic Society Clinical Practice Guideline. Am J Respir Crit Care Med 2024;209:24-36. [Crossref] [PubMed]
- Matta A, Chaudhary S, Bryan Lo K, et al. Timing of Intubation and Its Implications on Outcomes in Critically Ill Patients With Coronavirus Disease 2019 Infection. Crit Care Explor 2020;2:e0262. [Crossref] [PubMed]
- Papoutsi E, Giannakoulis VG, Xourgia E, et al. Effect of timing of intubation on clinical outcomes of critically ill patients with COVID-19: a systematic review and meta-analysis of non-randomized cohort studies. Crit Care 2021;25:121. [Crossref] [PubMed]
- Hernandez-Romieu AC, Adelman MW, Hockstein MA, et al. Timing of Intubation and Mortality Among Critically Ill Coronavirus Disease 2019 Patients: A Single-Center Cohort Study. Crit Care Med 2020;48:e1045-53. [Crossref] [PubMed]
- Crimi C, Pierucci P, Renda T, et al. High-Flow Nasal Cannula and COVID-19: A Clinical Review. Respir Care 2022;67:227-40. [Crossref] [PubMed]
- Grasselli G, Calfee CS, Camporota L, et al. ESICM guidelines on acute respiratory distress syndrome: definition, phenotyping and respiratory support strategies. Intensive Care Med 2023;49:727-59. [Crossref] [PubMed]
- Pandya A, Kaur NA, Sacher D, et al. Ventilatory Mechanics in Early vs Late Intubation in a Cohort of Coronavirus Disease 2019 Patients With ARDS: A Single Center's Experience. Chest 2021;159:653-6. [Crossref] [PubMed]
- Hyman JB, Leibner ES, Tandon P, et al. Timing of Intubation and In-Hospital Mortality in Patients With Coronavirus Disease 2019. Crit Care Explor 2020;2:e0254. [Crossref] [PubMed]
- González J, Benítez ID, de Gonzalo-Calvo D, et al. Impact of time to intubation on mortality and pulmonary sequelae in critically ill patients with COVID-19: a prospective cohort study. Crit Care 2022;26:18. [Crossref] [PubMed]
- Vera M, Kattan E, Born P, et al. Intubation timing as determinant of outcome in patients with acute respiratory distress syndrome by SARS-CoV-2 infection. J Crit Care 2021;65:164-9. [Crossref] [PubMed]
- Roca O, Messika J, Caralt B, et al. Predicting success of high-flow nasal cannula in pneumonia patients with hypoxemic respiratory failure: The utility of the ROX index. J Crit Care 2016;35:200-5. [Crossref] [PubMed]
- Attaway AH, Scheraga RG, Bhimraj A, et al. Severe covid-19 pneumonia: pathogenesis and clinical management. BMJ 2021;372:n436. [Crossref] [PubMed]
- Villarreal-Fernandez E, Patel R, Golamari R, et al. A plea for avoiding systematic intubation in severely hypoxemic patients with COVID-19-associated respiratory failure. Crit Care 2020;24:337. [Crossref] [PubMed]
- Yang X, Yu Y, Xu J, et al. Clinical course and outcomes of critically ill patients with SARS-CoV-2 pneumonia in Wuhan, China: a single-centered, retrospective, observational study. Lancet Respir Med 2020;8:475-81. [Crossref] [PubMed]
- Docherty AB, Mulholland RH, Lone NI, et al. Changes in in-hospital mortality in the first wave of COVID-19: a multicentre prospective observational cohort study using the WHO Clinical Characterisation Protocol UK. Lancet Respir Med 2021;9:773-85. [Crossref] [PubMed]
- Roth GA, Emmons-Bell S, Alger HM, et al. Trends in Patient Characteristics and COVID-19 In-Hospital Mortality in the United States During the COVID-19 Pandemic. JAMA Netw Open 2021;4:e218828. [Crossref] [PubMed]
- Menga LS, Berardi C, Ruggiero E, et al. Noninvasive respiratory support for acute respiratory failure due to COVID-19. Curr Opin Crit Care 2022;28:25-50. [Crossref] [PubMed]
- Ospina-Tascón GA, Calderón-Tapia LE, García AF, et al. Effect of High-Flow Oxygen Therapy vs Conventional Oxygen Therapy on Invasive Mechanical Ventilation and Clinical Recovery in Patients With Severe COVID-19: A Randomized Clinical Trial. JAMA 2021;326:2161-71. [Crossref] [PubMed]
- Perkins GD, Ji C, Connolly BA, et al. Effect of Noninvasive Respiratory Strategies on Intubation or Mortality Among Patients With Acute Hypoxemic Respiratory Failure and COVID-19: The RECOVERY-RS Randomized Clinical Trial. JAMA 2022;327:546-58. [Crossref] [PubMed]
- Tobin MJ, Laghi F, Jubran A. Caution about early intubation and mechanical ventilation in COVID-19. Ann Intensive Care 2020;10:78. [Crossref] [PubMed]
- Marini JJ, Gattinoni L. Management of COVID-19 Respiratory Distress. JAMA 2020;323:2329-30. [Crossref] [PubMed]
- Yamamoto R, Kaito D, Homma K, et al. Early intubation and decreased in-hospital mortality in patients with coronavirus disease 2019. Crit Care 2022;26:124. [Crossref] [PubMed]
- Polok K, Fronczek J, Artigas A, et al. Noninvasive ventilation in COVID-19 patients aged ≥70 years-a prospective multicentre cohort study. Crit Care 2022;26:224. [Crossref] [PubMed]
- Roca O, Caralt B, Messika J, et al. An Index Combining Respiratory Rate and Oxygenation to Predict Outcome of Nasal High-Flow Therapy. Am J Respir Crit Care Med 2019;199:1368-76. [Crossref] [PubMed]
- Zucman N, Mullaert J, Roux D, et al. Prediction of outcome of nasal high flow use during COVID-19-related acute hypoxemic respiratory failure. Intensive Care Med 2020;46:1924-6. [Crossref] [PubMed]
- Prakash J, Bhattacharya PK, Yadav AK, et al. ROX index as a good predictor of high flow nasal cannula failure in COVID-19 patients with acute hypoxemic respiratory failure: A systematic review and meta-analysis. J Crit Care 2021;66:102-8. [Crossref] [PubMed]
- Carrillo A, Gonzalez-Diaz G, Ferrer M, et al. Non-invasive ventilation in community-acquired pneumonia and severe acute respiratory failure. Intensive Care Med 2012;38:458-66. [Crossref] [PubMed]
- Thille AW, Contou D, Fragnoli C, et al. Non-invasive ventilation for acute hypoxemic respiratory failure: intubation rate and risk factors. Crit Care 2013;17:R269. [Crossref] [PubMed]
- Liu L, Xie J, Wu W, et al. A simple nomogram for predicting failure of non-invasive respiratory strategies in adults with COVID-19: a retrospective multicentre study. Lancet Digit Health 2021;3:e166-74. [Crossref] [PubMed]
- Grapsa E, Adamos G, Andrianopoulos I, et al. Association Between Vaccination Status and Mortality Among Intubated Patients With COVID-19-Related Acute Respiratory Distress Syndrome. JAMA Netw Open 2022;5:e2235219. [Crossref] [PubMed]
Cite this article as: Ekkapat G, Sirilaksanamanon P, Theerasuwipakorn N, Phatharamalaipong N, Klarod S, Phannajit J, Sae-Phua V. Timing of intubation and clinical outcomes in critically ill COVID-19 patients with respiratory failure. J Emerg Crit Care Med 2024;8:20.